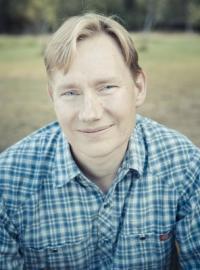
Associate Member
Contact Information
- brumer@msl.ubc.ca
- Office: MSL 325
- Office Phone: 604-827-3738
- Lab: MSL 263
- Lab Phone: 604-827-3759
My Links
Associate Member
Contact Information
My Links
Robert Francis Scagel (1921–2018)
Obituary by Louis Druehl1, Bamfield, BC, Canada, January 2019
Robert Francis Scagel’s (1921-2018) career’s journey began in Ontario where he fine-honed his business skills at a business college. In time he moved to British Columbia and a variety of business-related jobs, including the Boeing Manufacturing plant in Richmond, BC, where Hurricane fighter aircraft were made. A mystic transition found him at the University of British Columbia (A.B. 1947, M.A. 1948) and at Phykos’ Door. Next, U.C. Berkeley and a Ph.D. under Associate Professor George Frederik Papenfuss’s tutelage in 1952.
A joint Oceanography/Botany appointment brought him back to UBC and his second career tract: Academic. The story goes that Dr. Papenfuss assigned the first efforts to study the algal macroflora of the eastern Pacific Coast to himself (California north to the Canada-US border), and north of the 49th parallel to Scagel. In this role he synthesized seaweed literature relevant to the local flora, produced a world class herbarium, based on ten years of intense collecting from Attu, Alaska to Terra del Fuego, Chile, leap-frogging Papenfuss’s domain, and supported numerous graduate students, postdocs, and research associates.
Dr. Scagel developed a ‘Synthetic Approach’ to understanding seaweed distributions, relating known seaweed distributions to coastal oceanographic conditions. This approach resulted in predicting kelp refugia in tropical deep-waters (Graham, et al. 2007. PNAS 104: 16576-16580). I believe this research was important to his being elected a Fellow of the Royal Society, Canada.
Outside of the lab, he guided the Botany Department as Head from 1972-1985. During this period there were several faculty members with algal interests: (J. Stein – freshwater, K. Cole – cytogenetics, B. Tregunna – physiology, T. Bisalputra – ultrastructure, R. de Wreede – ecology, R. Foreman – ecology, cultivation, F. J. R. Taylor – phytoplankton, P. J. Harrison – phytoplankton/seaweed physiology, P. G. Harrison – eelgrass ecology, Luis Oliveira – ultrastructure, Michael Hawkes – red algal systematics, life histories). Wrote David Garbary of this assemblage, “I have always looked back to my years at UBC with pride. In the 70s and early 80s Bob made that institution a world centre for phycology, and I am humbled to have been a part of that.” Dr. Scagel was editor2 of Phycologia, 1969-1971, and on the organizing committee for the 1st Phycological Congress, 1982.
Following his retirement in 1986, he slipped into his third career, visual artist (https://ginkgostudio.weebly.com/) where he quickly established his exploratory artistic excellence. During his last years, he lived with his son, Rob. His vision, hearing and mobility were ravaged, but his mind remained sharp.
My Phykos Door was opened when Professor Scagel discovered me, pouting, on WestBeach, WhidbeyI., WA., contemplating a career in the gas industry (I would walk gas lines looking for sick plants). He was putting seaweeds in a bucket and I asked him, “What are you doing?” He replied, “Collecting seaweeds.” And I said, “Oh, and who are you?” “Bob Scagel,” he replied. After a few more inane exchanges, Scagel said, “Would like to study at UBC?” Thank you, Bob, thank you for everything. R.I.P.
1Dr. Druehl was a Ph.D. student with Prof. Scagel; completing his thesis, ‘On the taxonomy, distribution, and ecology of the brown algal genus Laminaria in the northeast Pacific’, in 1965.
2Dr. Scagel was also Editor of Syesis (published by the BC ProvincialMuseum) from 1968–1975 (Vols. 1–8).
******************************************************************************************
Further information on Prof. Scagel’s career can be found in Taylor (2017) A History of the University of British Columbia Botany Department 1908-2015.
Images and selected publications (courtesy of Mike Hawkes).
Figure 1
Figure 2
Figure 3
Figs. 1, 2 & 3. The canopy-forming kelps Macrocystis (1 & 2) and Nereocystis (3).
In a paper published just after he completed his B.A. degree at UBC, Scagel (1947) reported on seaweeds, especially ‘Giant kelp’ (Macrocystis pyrifera) and ‘Bull kelp’ (Nereocystis luetkeana), in the vicinity of HardyBay, on the NE coast of Vancouver I. This publication was based on field work carried out by Scagel at a station on DeerIsland from July to September 1947. The report focused on the growth, effect of harvesting, and conservation of these important seaweeds.
Figure 4
Fig. 4. Whidbeyella cartilaginea distribution as of 2019.
This extremely rare red seaweed was first described by Setchell and Gardner (1903) from a single drift specimen on the west coast of Whidbey I., WA. Scagel (1962) published the first account of the reproductive anatomy of the monotypic genus Whidbeyella, based on a collection from near Smith I., WA. Scagel also reported on the largest known specimen of Whidbeyella (lower left corner of Fig. 4) collected by Mike Neushul at Hein Bank, WA and photographed by Shirley Sparling while at the University of Washington’s Friday Harbor Labs, San Juan I.
Figure 5
Fig. 5. Scagel (1971) reported the first occurrence of the brown alga, Dictyota binghamiae, in British Columbia. This widespread genus is usually found in warm temperate to tropical waters.
Figure 6
Fig. 6. Bob Scagel and students collecting seaweeds at Kiix-in1 (pronounced KEE-shun in the Ohiat language), Barkley Sound, Vancouver I., BC, 10 May 1974. Kiix-in was once the capital community or main village of the Huu-ay-aht group of the Nuu-chah-nulth First Nation. Declared a National Historic Site in 1999, it is close to the Bamfield Marine Sciences Centre. Scagel (1973) published the first synopsis of benthic marine plants in the vicinity of Bamfield.
Figure 7
Fig. 7. Bob Scagel and Jean Feldmann (France) collecting seaweeds at Point No Point, near Sooke on the SW coast of Vancouver Island in 1959.
In an email (9 Dec. 2002), Scagel told the story of this field trip to some former students and colleagues (Widdowson, Druehl, Hawkes, Gabrielson, and Lindstrom). It is worth quoting here …… “I first met Feldmann in 1955 when I visited him in Paris and in the summer of 1959 as part of the International Botanical Congress in Montreal when I led a small field trip of several days duration to Vancouver Island (Sooke and Port Renfrew). That field trip was unique, because it comprised only five of us – fitting into one station wagon: Feldmann, Yamada, Helen Blackler (from St. Andrews University), Ron Taylor from New Brunswick and George (whose surname I have forgotten) from San Francisco. We stayed several nights at the Point No Point Motel in three separate cottages. Helen Blackler by herself in one cottage; Feldmann and Yamada in one cottage; and myself and the other two in a third. I often thought afterward that Feldmann and Yamada must have made a curious pair: At that time Feldmann spoke very little English and Yamada spoke very little English! The following year Yamada came to Friday Harbor where we shared in offering the course in “algology”. That field trip was also unique in one other sense: Helen Blackler had been visiting a relative in Victoria prior to the field trip and was just recovering from the “flu”. Our first night at Point No Point she was ill and lost one of her dentures (upper or lower, I don’t recall) down the John. Recovery was impossible, so I spent part of the next three days driving her back and forth to a dentist in Sooke. By the time we left, she probably went back to Scotland with one of the finest sets in Scotland. That field trip was also unique in that, courtesy of the Canadian Navy, we made a side trip from Victoria to Friday Harbor (and a dredging trip on the Hydah). We made quite an impression on the Friday Harbor residents and Marine Station with our naval escort.”
Figure 8
Fig. 8. Tom Widdowson (then doing his Ph.D. with Scagel), Y. Yamada, Ron Taylor (back), George ? (front), H. Blackler, and J. Feldmann at Botanical Beach, 1959. Continuing Scagel’s story of this field trip (email 9 Dec. 2002)….. “Our trip to Botany Beach at Port Renfrew was also noteworthy. In those days, as Tom will no doubt recall, we parked in the village of Port Renfrew and hiked over the remnants of the old plank road. During the field trip, Feldmann wore a pair of open plastic sandals, one of which he lost on the way back over the plank road. When the field trip ended we provided space in the Botany Department [at UBC] for those who had specimens to mount to do so. Julie [Celestino] changed the blotters and completed the drying and we mailed the specimens to them. In the meantime, Feldmann’s sandal was recovered, and we mounted it on a herbarium sheet and sent it back to Paris with his specimens.”
Figure 9
Fig. 9. Four distinguished phycologists. Sapporo, Japan, Aug. 1971. Scagel (email 9 Dec. 2002) also provided the story for this photo ….. “This picture was taken by Jun Tokida during the 7th International Seaweed Symposium in Sapporo. I had met Tokida and Yamada when I visited Sapporo in 1957. Tokida, whose English was quite good, came from Hakodate to Sapporo to meet me. When he met me at the airport he was holding a big sign that said “welcome Dr. Scagel”. He had borrowed the chauffeur and limousine of the President of Hokkaido University. The following day they took me on a field trip to the marine station at Oshoro (I think) in the shadow of Mt. Yendo – the first time I ever went on a field trip in a chauffeured Cadillac! The mountain nearby was apparently named after Yendo after his return from the University of Chicago and he was allegedly responsible for introducing skiing to Hokkaido on his return to Japan. In 1971 Yamada was in failing health and was living with his son in Sapporo. Tokida took the four of us (in the photograph) by taxi to visit Yamada. Those in the picture are left to right: seated Jean Feldmann and Y. Yamada; standing RFS and G. F. Papenfuss.
Figure 10
Fig. 10. The first five Editors of the journal Phycologia: Kay Cole, Robin South, Bill Woelkerling, Robert Scagel, and Paul Silva pose for a photo during the 13th International Seaweed Symposium held at UBC, Vancouver, Aug. 1989.
Figure 11
Fig. 11. Bob Scagel and Olivia Lee at the UBC Herbarium 100th Anniversary Celebration, 29 Feb. 2016.
***************************************************************
1Formerly known as Execution Rock
Photo Editing and Captions:
Michael W. Hawkes
Photo Credits:
Figs. 1-6 and the portrait of RF Scagel on the 1st page: Michael W. Hawkes.
Figs. 7-10. Photos courtesy of RF Scagel. Fig. 7 Photographer unknown. Fig. 8 photo by RF Scagel, Fig. 9 photo by Jun Tokida, Fig. 10 Photographer unknown,
Fig. 11. UBC photographer.
Historical Video (1957):
A 1957 educational TV series called ‘The Living Sea’ with Dr. Ian McTaggart-Cowan (former Head of Zoology Dept., UBC). Dr. Robert F. Scagel makes a guest appearance in this 1/2 hr episode:
https://exhibits.library.uvic.ca/uploads/spotlight/resources/videoupload/url/3424/3422.mp4
Thank you to Dr. Scagel’s son, Rob, for bringing this video to my attention (MW Hawkes).
Selected Early Publications
Scagel, R. F. 1947. An investigation of marine plants near HardyBay, B. C. Provincial Department of Fisheries, Victoria. No. 1. 70 pp. 26 figs.
Scagel, R. F. 1957. An annotated list of the marine algae of British Columbia and northern Washington. National Museum of Canada, Bulletin 150: 1‑289. 1 fig.
Scagel, R. F. 1961. The distribution of certain benthonic algae in Queen Charlotte Strait, British Columbia, in relation to some environmental factors. Pacific Science 15: 494‑539. 51 figs.
Scagel, R. F. 1962. A morphological study of the red alga Whidbeyella cartilaginea Setchell et Gardner. Canadian Journal of Botany 40: 1217‑1222. 14 figs.
Scagel, R. F. 1963. Distribution of attached marine algae in relation to oceanographic conditions in the northeast Pacific. Pp. 37‑50. 11 figs. In Dunbar, M. J. (ed.), Marine distributions. University of Toronto Press.
Scagel, R. F. 1964. Some problems in algal distributions in the North Pacific. Proceedings of the International Seaweed Symposium 4: 259‑264.
Scagel, R. F. 1966a. Marine algae of British Columbia and northern Washington. Part 1. Chlorophyceae. National Museum of Canada, Bulletin 207: 1‑257. 49 pls.
Scagel, R. F. 1966b. The Phaeophyceae in perspective. Oceanography and Marine Biology Annual Reviews 4: 123‑194. 1 fig.
Scagel, R. F. 1967. Guide to common seaweeds of British Columbia. British ColumbiaProvincialMuseum, Handbook No. 27, 330 pp. 141 figs.
Scagel, R. F. 1971 [1972]. The brown alga Dictyota binghamiae J. Ag. from British Columbia and northern Washington. Syesis 4: 261. 1 fig.
Scagel, R. F. 1973. Marine benthic plants in the vicinity of Bamfield, Barkley Sound, British Columbia. Syesis 6: 127‑145. 1 fig.
Scagel, R. F., R. J. Bandoni, J. R. Maze, G. E. Rouse, W. B. Schofield, & J. R. Stein. 1982. Nonvascular plants: an evolutionary survey. Wadsworth Publishing Co., Belmont, California.
Scagel, R. F., R. J. Bandoni, J. R. Maze, G. E. Rouse, W. B. Schofield, & J. R. Stein. 1984. Plants: an evolutionary survey. Wadsworth Publishing Co., Belmont, California.
Reprinted with permission from the Botanical Electronic News (click here to see original article on BEN)
Neil Towers, a much respected scientist and Professor at the University of British Columbia, passed away on November 15th, 2004 in Vancouver. He was 81. Predeceased by mother Kathleen and brother Desmond, Neil will be lovingly remembered and sadly missed by his wife Elizabeth and his eight children. He will be greatly missed by colleagues, students, and friends at the University of British Columbia (Botany Department) and around the world.
UBC Emeritus Professor of Botany Neil Towers was well known nationally and internationally for his outstanding record of pioneering and sustained research in botany and phytochemistry.
He was born in Bombay, India and grew up in Burma, where his interest in the natural world began. He often spoke of his childhood spent collecting poisonous snakes and other curiosities in the forests near his home.
After time in the Royal Indian Navy Volunteer Reserve, and a stint as a liaison officer in Bath, England, he was awarded an Ajax scholarship to study in Canada. He obtained his B.Sc. and M.Sc. from McGill University, and his Ph.D. in 1954 from Cornell University. After academic appointments at McGill and the NRC in Halifax, he was recruited to UBC, where he served as Head of the Department of Botany from 1964-71, a period of great expansion of the Department. After 1971, he devoted his full energies to his successful career in research and teaching, which he continued as an emeritus faculty member from 1989 until his death.
Neil was a Fellow of the Royal Society of Canada, from whom he received the Flavelle Medal in 1986. He received numerous research awards and prizes over his career. Most recently, he was awarded the Pergamon Phytochemistry Prize in 2000, and in 2001 was recognized by ISI as one of UBC’s (and the world’s) most highly cited scientists. He published more than 425 papers and book chapters, starting with a 1953 paper in Nature.
Neil was charming, funny and an excellent raconteur. Lab parties at his Vancouver home often ended late at night, with the room cleared to make space for dancing to his favorite Django Reinhardt and latin music. He had an open door policy, and always welcomed office visits by students or whoever was interested in talking about plants and chemistry. He traveled extensively to collect plants worldwide, mainly in the tropics, and he returned from a trip to Peru only this last summer. Many in the Botany Department at UBC will remember the photos and artifacts from his travels that decorated his office.
Neil loved what is now called biodiversity: the shapes and colours of plants and insects, and the variations of chemical structures found in nature. His fields of study included medicinal phytochemistry, ethnopharmacology, photobiology, chemical ecology relating to plants, fungi and insects, and biotechnology of plant cell and tissue cultures. He conducted important early studies on phenolic metabolism in plants, and on the interaction of light with phytochemicals to produce toxicity. His lab investigated the chemistry and antibiotic activity of many plants native to British Columbia, including those used in traditional medicine.
Neil was also a great teacher of young scientists in Canada and elsewhere, and many of his graduate students and postdoctoral workers went on to establish their own labs. Perhaps his greatest contribution to science was through this role as mentor. A student in his lab couldn’t walk by his office door without being called in to discuss a new paper or a new idea. It is through his infectious enthusiasm for science and the natural world that the spirit of Neil Towers lives on.
WHAT ARE YOU GOING TO BE WHEN YOU GROW UP, DAD?
From: G.H. Neil Towers. 2001.
Some Memories of a Budding Scientist in North America (1946-1965) Phytochemical Society of North America News, October 2001, p. 3-5. (Phytochemical Society of North America webpage)
I grew up in Myanmar (formerly Burma) when it was a British colony. My parents sent me to boarding schools around the country run by Christian brothers who sadly lacked an interest in the natural sciences, particularly natural history. Living and traveling as a schoolboy in perhaps one of the most beautiful tropical countries on this planet, I developed a craze for natural history. I collected snakes, beetles, butterflies, dissected animals for parasites and tried to identify plants from books. It was a happy boyhood. On reflection I think I was lucky not to have lived in our computer and television age. I did not see a television program until I was about twenty two! I spent all of my holiday time escaping prayers and wandering through the enchanting countryside exploring nature. I was spellbound by the travels, adventures and ideas of Darwin, Wallace, Bates and many other famous explorers. That is exactly what I wanted to be. World War II intervened.
I came to Canada on a scholarship for ex-naval officers at the end of the war. I had many adventures during the war, quite a number of which would have been called unforced errors of life were they to be compared to a game of tennis! Having escaped from the Japanese and winding up in England and then Canada, my life changed and I was suddenly plunged into the cloisters of academia. My sunny days of adventure were over – perhaps forever.
I was saddened to find that there were very few enthusiastic natural historians in this new life in a university. My fellow undergraduates in fact never seemed to have had time to talk about the excitement of biology they were so busy cramming for exams. I found out also that the world appeared to have been already explored by my arrogant zoology instructors and there was little new to discover other than to climb very tall moun- tain peaks or dive deep under the sea. I was an Honours Zoology student at McGill University in Montr,al at the time and was advised by zoologists that the secrets of the animal world really lay in the realm of statistics! Even genetics was all statistics according to them.
Botanists, in contrast, were fascinated by apparent trivia: they were excited by the shapes of leaves, the hairiness of plant structures (for which there are many unpronounceable names) the geometry of flowers and a phenomenon called 2N versus N. However these botanists seemed to love what they were doing and I was encouraged to join their ranks. They actually worked with their microscopes in the evenings. They suggested to me that the inner workings of plants e.g. how sugars are manufactured from a gas in light was irrelevant and for Heaven’s sake don’t spoil things by dragging chemistry into the picture in order to understand how a plant lives. Of course, electron microscopy, the role of nucleic acids, the nature of enzymes etc. were not even dreamed of at that time. Professor R. D. Gibbs, a feisty botanist at McGill, kindled my interest in plant chemistry. He was con- sidered a crank by other botanists as I found out later because he was fascinated by the chemical relationships between plants. In fact he was a chemotaxonomist at a time when chemists did not know the meaning of the word taxonomy and a botanist might have been embarrassed if accused of understanding anything about chemistry.
Here was a botanist who actually knew some phytochemistry and, Good Lord, this chap could actually draw chemical structures! We became good friends and I obtained an M.Sc. under his supervision. The research involved the chemotaxonomy of plant lignins and was published in Nature. Perhaps I was the first person at McGill to use the new technique of paper chromatography. Certainly, the chemists and biochemists at McGill seemed to be as yet unfamiliar with the use of this technology. Later on during a sabbatical leave with the enzymologist D.D. Davis at the University of East Anglia, I used to drink beer every afternoon with Dick Synge, one of the discoverers of paper chromatography, a Nobel Laureate, a seasoned beer drinker, and a very dangerous cyclist (after drinking of course). Curiously Gibbs suggested that paper chromatography would never work and that I should use fractional sublimation instead to separate my products of alkaline nitrobenzene oxidation, namely p-hydroxybenzaldehyde, vanillin and syringaldehyde. Clearly this was not good advice because his total NRC budget for the year was about $115! Gibbs was old fashioned by current standards. It was good for me. It made me more of an independent scientist. When I asked him if he would be kind enough to read a draft of my thesis he was astounded. “I am here to examine you Towers, not to help you. It is your thesis – not mine!” Nowadays, of course, there are many rules in Canadian universities to chaperone graduates in thesis writing so that in the end I feel we sometimes produce the well- known camel instead of the desired racehorse.
I went to Cornell for my Ph.D. studies with Professor F. C. Steward, a distinguished English plant physiologist who boasted that he had never taken a course in botany in his life. Needless to say he thought that he was the founder of botany. He had achieved fame for his work on ion accumulation in plants. His students called him the Golden Bantam because he was small, a fearless fighter, and rather arrogant. He once told me that he regretted the fact that dueling was no longer encouraged as a means of resolving departmental quarrels among faculty. He meant it!
Steward had become interested in the use of paper chromatography for separating and identifying the many unidentified non-protein amino acids in plants. My Ph.D thesis was concerned with designing new methods for the identification of alpha-keto acids in plants. Quite boring actually. It was a wonderful period of study, however, because Steward had attracted extremely knowledgeable postdocs, such as John F. Thompson, and clever graduate students to his lab. He was also a research supervisor who was so busy chasing research dollars that we had complete freedom in our own programs. I think that this is still the case in many universities.
At Cornell I took Botany and Biochemistry and a course on enzymes by J. B. Sumner. After many years of tedious research Sumner had discovered that the fewer steps involved, the better were his yields of the hydrolytic enzyme urease which he was studying in Canavalia ensiformis (Jack bean). In fact, he discovered one day that a 32% acetone extract heated to about 60 deg. C, filtered overnight through Whatman paper into a graduate cylinder, and placed in a refrigerator yielded a precipitate which, when examined under a microscope, was found to consist of “octahedral” crystals. The crystals had tremendous urease activity. Repeated analyses showed that it was a protein. This was in 1926. Sumner wrote in his lab notebook about this momentous day: “That night I slept but little”. At that time of course the true nature of enzymes was unknown and the leaders in the field, among them the very famous German biochemists, Willstatter and Waldschmidt-Leitz, refused to acknowledge that a 26-year-old American had actually isolated an enzyme and proven that it was nothing more than a protein. His discovery was treated with some ridicule which unfortunately made him rather bitter. Four years later when Northrup crystallized the proteolytic enzymes pepsin and trypsin from animal sources at the Rockefeller Institute and showed that they are also proteins, Sumner’s achievement was acknowledged – they shared a Nobel prize. We had the privilege of repeating Sumner’s work in our laboratory course and even of recrystallizing urease. Of course, like Sumner, we made the entry “That night I slept but little” in our laboratory notebooks.
I was offered a job as Assistant professor in the Botany Department at McGill and assigned to teach plant anatomy, plant physiology, plant biochemistry and help run introductory botany labs. I inherited an old physics lab which could only be accessed through a men’s urinal, a bit of an annoyance to my women graduate students. After four years of working in this “lab” we discovered an open pool of about 40 kg of mercury under the wooden floor. It must have been “lost” by the physicists during their war research years. Also contributing to the poor working conditions were the feral cats that had taken up residence in the dark corners of this medieval set of rooms, occasionally emerging to produce a litter of young kittens on our chromatograms which had to be stored on the floor. The lab was cold and we often had to use gloves and overcoats to stay warm during the winter months. At that time, university startup money for research was unthinkable. Besides, there were tons of microscopes and herbarium sheets lying around. What more could a botanist want? Roy Waygood, the plant physiologist at McGill was most encouraging, allowing me access to his lab equipment and his knowledge of plant physiology and biochemistry.
Among the many wonderful graduate students in my lab was our illustrious PSNA [Phytochemical Society of North America] stalwart Ragai Ibrahim. Seichi Yoshida of Tokyo Metropolitan University also joined me as a postdoc. Later his student, Minamikawa, joined my lab and much later on Minamikawa’s student, Etsuo Yamamoto, came to my lab as a postdoc. That’s three generations of great Japanese phytochemists! We spent a lot of time making 2D chromatograms of plant extracts, cutting out spots, eluting them, and so on. I remember my eight year old son spending an afternoon in my lab. After watching me for half an hour he asked “What are you going to be when you grow up, Dad?” It seems that in his eyes I have never really grown up.
I was delighted to learn about the Birch and Donovan hypothesis in relation to flavonoids. Instead of being neatly derived (on paper) from two hexoses and a triose according to Geissman and Hinreiner, they now appeared to be derived from a hydroxycinnamate and acetate! We resolved to test this with the dihydro-chalcone glucoside, phloridzin. Alas! We were beaten by Neish’s group at the National Research Council (NRC) of Canada in Saskatoon who proved this hypothesis with quercetin, and Grisebach’s group in Germany who proved the hypothesis with an anthocyanin. It seems silly now but we were dreadfully disappointed not to have been the first to have proven that Birch and Donovan were right.
I spent a summer at the NRC laboratories in Ottawa with D.C. Mortimer and Paul Gorham, learning radiotracer techniques and carrying out 14C photosynthesis studies. The following summer I spent with Stewart “Coumarin” Brown and Arthur Neish at the NRC laboratory (then called the Prairie Regional Laboratory) in Saskatoon studying coumarin biosynthesis. When I returned to McGill at the end of the summer Ibrahim and I prepared twodirectional chromatograms of the phenolic acids from a range of plants. Sprayed with diazotized nitroaniline or diatized sulfanilic acid they gave a range of beautiful colors. We had a special room set up with these large chromatograms adorning the walls for participants of the IXth Botanical Congress which was held at McGill, the Universit, de Montr,al, and Sir George Williams College (now Concordia University) that year. These chromatograms were works of art and admired by all who visited us.
After enjoying more than nine years at McGill I was invited by Art Neish to head up the Plant Biochemistry section of the NRC’s Atlantic Regional Laboratory in Halifax, Nova Scotia to which he had been appointed Director. Neish was considered to be one of the outstanding phytochemists in Canada and I was delighted to join his institute as I had a great admiration for him as a scientist and also because I was jointly appointed as an Associate Professor to Dalhousie University in Halifax where Neish and I taught a course in comparative biochemistry. My graduate students at McGill accompanied me there and had the advantage of working in the well-equipped NRC labs and alongside distinguished Canadian scientists in chemistry (Gavin McInnis) and biochemistry (Leo Vining). We published many papers especially on the biosynthesis of interesting lichen compounds as well as on comparative phenylpropanoid metabolism in lycopods and fungi. We showed clearly that L-tyrosine is metabolized quite differently from L-phenylalanine, especially in vascular plants. Tyrosine is metabolized to acetate and its derivatives when introduced into plant tissues and phenylalanine is the gateway to phenylpropanoid metabolism. We also identified a new cyanogen from Taxus and studied its biosynthesis showing that both the nitrogen and carbon are derived from L-phenylalanine. We discovered psilotin, a glucoside derived from ahydroxycinnamate and one equivalent of acetate, in the primitive ferns Psilotum and Tmesipteris.
I next moved to the University of British Columbia in Vancouver as Head of Biology and Botany, an administrative position which tore me away from thinking time and plunged me into the petty life of administration in a then impoverished Canadian university. After five years, a sabbatical leave in England where I studied enzymes with D.D. Davies at the University of East Anglia, convinced me to resign as Head and settle down again to research and teaching. As most biology students at our universities do not enjoy chemistry my classes were small and were therefore a great pleasure to teach. Many more graduate students and postdocs passed through my research program and it would take many more pages to describe our further achievements in phytochemistry.
Profile and tribute by Jack Maze and Jim Pojar
Katherine I. Beamish, Kay, was brought up on a Manitoba farm and moved to Burnaby, B. C. in her teens. She trained as an elementary school teacher at the Vancouver Normal School and taught in elementary schools throughout the 1930s until World War II. During the war Kay enlisted in the women’s division of the Royal Canadian Air Force and was based in Halifax and Newfoundland through the years of the Battle of the Atlantic. She served in the air ferry command that flew bombers across the North Atlantic. After the war, she obtained a B. S. Agriculture and M. Sc. in Plant Sciences at UBC. She then did a Ph.D. in plant taxonomy at the University of Wisconsin. Kay was invited back to the Botany Department at UBC, in part to help re-establish the herbarium. Through neglect during the Great Depression and World War II, several of its early plant collections by pioneer botanists had been virtually destroyed by beetles. She added greatly to the UBC herbarium collection and helped restore confidence in its usefulness. The Botanical Garden at UBC had dwindled to a few taxonomic beds, and Kay also helped with its restoration. She remained with the UBC Botany Department until retirement. In the 1950s and ‘60s, Kay and others assisted Vladimir Krajina in the development and passage of the B.C. Ecological Reserve Act. Kay was very active in the Vancouver Natural History Society and was the inspiration for the VNHS’s Kay Beamish Award for Achievement in Nature Education, established in 1995. She died peacefully in Crofton on February 4, 2003 at the age of 90.
Much of this information came from the Vancouver Natural History Society’s journal Discovery, Spring 2003, vol. 32 no. 1, pp. 8-12.
Jim Pojar remembers Kay Beamish
Dr. Katherine Beamish was one of the first Canadians I met, when in 1970 I came to Vancouver, for graduate studies in the Department of Botany. She made an immediate favourable impression on me, and has had a lasting effect on my life. I very soon realized she was a real botanist, and was a kind but no-nonsense person who I instinctively knew I must not disappoint. She became my supervisor, and guided me through the unpredictable academic terrain with care and skill. Every Friday, for three years, she sat down with me and discussed what I had been working on that week, what progress I had made on the thesis. Few Ph.D. supervisors spend that much time with their grad students. Her diligence with me was especially noteworthy because I wasn’t working on any of her favourite projects or species (saxifrages and shootingstars), nor did my research directly benefit her program. She was also remarkably tolerant of my headstrong and at times stubborn and smart-alecky behaviour. No doubt she had seen that sort of thing before. Looking back on those days, I recall that there was often a glint of amusement in her eyes, at some of my antics.
But we had more than just a student-professor relationship. Early on, it became clear that we both really liked going out in the field, exploring and botanizing and doing natural history. Field botany was our shared passion, and later on the Vancouver Canucks, who have been much less rewarding. It was Kay Beamish who first took me to Lighthouse Park, to Ladner Marsh, Camosun Bog, Burns Bog, Mill Hill, Thetis Lake Park, Long Beach, Liumchen Ridge, Blackwall Meadows and Mt. Frosty in Manning Park, among others. Those trips and places had a profound effect on me, a Minnesota boy new to mountains and the sea. I was also mightily impressed that this woman over twice my age could go uphill like a mountain goat.
Kay Beamish introduced me to Platanthera dilatata, Dodecatheon jeffreyi, Carex macrocephala, Saxifraga (now Micranthes) rufidula, Dicentra uniflora, Mimulus alsinoides, Larix lyallii, and many other marvels. I could go on and on, and we did, on some splendid extended field trips. Two in particular I remember. A collecting trip, with Jack Maze and some other students, to northern California—a whole new flora, where we ‘baled hay’ with the best of them. And an expedition up the just-completed Stewart-Cassiar road (now Highway 37), searching for plants and potential ecological reserves, with Keith Wade, Art Guppy, and George Otto. The northern bush was thrilling. We explored, hiked, bushwhacked, camped out in tents, collected and pressed plants well into the evening in the extended daylight, and had convivial times around the campfire.
I remember Kay Beamish most fondly as a mentor, my guide to a new world. I remember how sometimes in her office she would cock her head and get a faraway look, thinking perhaps of a past botanical ramble. Or how she would stand, hands clasping elbows, while gazing from some Chilliwack Valley peak towards Mt. Baker. She was a kind, generous, and good-humoured person who spoke no ill even of unpleasant people; at most she would grimace and shudder with exasperation. I am thankful that I met her, became her student and friend, was inspired by her, and settled in her beautiful province and country.
February 12, 2003
Image and write-up by Nivretta Thatra
As of summer 2022, Dr. Alex Moore (she/they) is an Assistant Professor co-appointed at UBC Botany and the Faculty of Forestry. We are thrilled to be working with them!
Moore’s research programme currently focuses on predator-prey interactions in mangrove forests throughout the tropics and will begin to include restoration projects along the coast of BC. These are incredibly important, timely research goals, keeping in form with Moore’s previous experience working as a postdoctoral fellow with the American Museum of Natural History in New York.
Given that description, seasoned scientists are likely to have a rough idea of the kinds of decisions and career transitions involved in Moore’s academic timeline. But for younger, newer scientists at the beginning of their journey, Moore’s path might seem hard to parse.
Read on to learn what Moore wants to share with young biologists — about their career path, how they learned research skills, and how work can align with community values.
To an outsider, it can seem like Dr. Moore has been on a linear, well-planned timeline to studying ecological conservation.
Moore elaborated on their timeline by stating that if someone had asked them ten years ago about where they would be now, they wouldn’t have been able to give a good answer. While they likely would have been able to envision themselves doing work related to conservation and the environment, they never would have guessed that they would be faculty.
“Each step along my academic timeline informed what I felt good about and what I didn’t feel good about doing in terms of what I wanted to do next,” they said.
Focusing on what was right in front of them – not what was ten years into the future – allowed Dr. Moore to be an intelligent and disciplined academic, and also gave them the space to figure out if they were enjoying the work. “At the beginning of my PhD I didn’t know what I’d be doing at the end of my PhD. All I was thinking about was, ‘I’m going to do my PhD,’” said Moore.
In fact, Dr. Moore’s path involved a few twists and turns. Check out the graphic above!
As an undergraduate, Moore started off as a chemistry major. It quickly became apparent that even though they earned high grades in the subject, they hated doing the work. Prompted by a sense of unease, they took time to self-reflect. When they thought about what they enjoyed doing and what they were drawn to, some themes arose: nature, spending time outdoors, and encouraging sustainable behaviors. Moore transitioned into the Ecology and Evolutionary Biology (EEB) major because the degree fit more of their interests.
They then did their Master’s degree in the same program (EEB), picking up key knowledge in conservation genetics and the transferable skill of lab work by working with a species of freshwater snails.
Learning wet-lab research skills was something Moore had to do on-the-fly while in their Master’s program.
“I had to do a lot to catch up to where everyone else was already starting,” said Moore. “People talk about the difficulty of getting into programs, and they also talk about the challenge inherent in academic spaces. But there is less conversation around where people think the starting line is, and whether or not you’re already at that starting point.”
Eventually, Moore realized they were not drawn to lab work. “I knew I was into conservation because I knew of my own personal connections to natural spaces and I knew that I wanted those spaces to persist into the future, but I didn’t really know how I wanted to fit into the field of conservation.”
While taking courses as a Master’s student, a class taught by Dr. Bradley Cardinale really caught Moore’s interest; the course examined the relationship between biodiversity, and ecosystems, and how people value and interact with natural systems. Moore was excited by how these topics prompted a diverse range of people to care about the science behind conservation. They carried this passion forward by incorporating species interactions and ecosystem services into their PhD research. During their PhD, Moore focussed on wetland ecosystems restoration in Connecticut. Instead of the more typical approach to trying to restore the physical parts of the system, such as nutrient availability via waterways, Moore incorporated the biological components of the system, such as interactions between predators and prey.
Moore’s PhD work showed that healthy ecosystem recovery is more likely when biological components of systems are incorporated into restoration. As a postdoctoral fellow, Moore expanded on this work by working on coastal mangrove restoration in the Pacific Islands, where they began to see the importance of traditional ecological knowledge and local environmental stewardship for ecosystems. These areas remain key to Moore’s current research.
However, Moore’s time as a PhD student also brought up some dislikes. They did not feel positively about the structure of academia, nor did their values align with the intense pressure to constantly publish. When pursuing postdoctoral opportunities, Moore decided to look for the opportunity to do “meaningful, impactful work that also supported equity, diversity inclusion for different kinds of communities of people.”
“The reason I took the postdoc position at American Museum of Natural History was because research was only one part of the role. The other part of my role was in teaching students from New York City who typically don’t have access to mentorship opportunities, and being able to do this spoke to the elements I felt were missing in my time in academia,” said Moore.
Moore’s journey through academia helped them identify the aims of the Inclusive Conservation Lab that they started when they arrived at UBC; they focus on applied community and ecosystem ecology research at various scales while incorporating the needs of local communities.
We concluded by asking Moore a few questions:
What would you say to a young scientist who doesn’t feel at home in academia?
Answer: I don’t know if it’s possible to really feel at home in an institution, and if it is possible, then it means that you’re aligned with whatever the institution is aligned with and maybe those alignments are problematic. I think what is possible is to feel at home in the community you decide to build within those spaces. When I was deciding where to do my PhD, a big element of my decision-making process was thinking through whether I would be in a space where I felt like I could have community. I had a terrible, terrible time in my Master’s program because I was brought into a non-inclusive space as a student in a non-traditional pathway; there were faculty members who didn’t think I belonged there or who weren’t convinced of the quality of my work.
While doing my PhD, I found home in people. You’ll always enter into an institutional culture that doesn’t align 100 per cent with your personal needs, but I think you can find trustworthy people that align with what makes you feel safe.
In your view, how did graduate school differ from the undergraduate experience?
Answer: While looking at graduate programs I was really aware of having to obtain financial support, the qualifying exam process, and coursework expectations. These all differ between institutions. I ended up choosing a program that felt right for me — my course load was low and the qualifying exam process was more involved but not as intense as other programs.
A challenge especially for young people coming into graduate spaces after the K-12 and undergraduate experience, where other people have structured your time for you, is being given the freedom to structure your own time while also being expected to complete a large scale research project. One thing you can do to establish some structure is to meet at the right intervals with your advisor. That interval, for me, was twice a month or once every couple weeks.
What is a commonly held belief about being a scientist that you want to dispel?
One of the big myths about what it takes to be a scientist is that you have to be “inherently” smart, that you have to be “inherently gifted”, or that you have to get the best marks on every standardized test you’ve ever taken.
Those things do not actually serve as metrics of whether or not you can be a good scholar. I don’t think that a certain background or mindset should disadvantage a person from being able to participate in this kind of scholarship, and I truly do think anyone could do this type of work with the right kind of support and with the right mentorship. I want more people to believe this!
Check out more of Moore’s work:
For Dr. Kaitlyn Gaynor (she/her), Assistant Professor in UBC’s Departments of Botany and Zoology, being able to do research was a key moment of transition in her journey to becoming a scientist.
“I had spent so much time in school reading other peoples’ work. When I began exploring research opportunities in undergrad, I realized that I could be the person that was creating knowledge and writing papers, as opposed to just being a consumer. It was really empowering,” said Gaynor.
Learning that, we got curious about what came before and after Gaynor’s pivotal insight. How did Gaynor get involved in research in the first place? How did her early research experiences lead her to her current interests in the effects of human disturbance on wildlife and to working with us at UBC Botany?
On behalf of young scientists, we asked Gaynor to help demystify her path as a way of offering advice to those who are new to research. The answers are summarized in the graphic above — but the insights don’t stop there. Read on to learn more!
At the beginning of undergrad, Dr. Gaynor didn’t know much beyond the fact that she wanted to do something in the sciences.
She felt a bit “like a kid in a candy shop” with the amount of options for classes that were available and decided to enroll in whatever she could that seemed interesting. This included classes in psychology, archaeology and ecology, and she even considered being a physics major.
Ultimately, one course adjacent to the field of ecology really caught Gaynor’s attention. The class, taught by Dr. Jill Shapiro, examined the history of the human species within ecosystems using the lens of human evolution. Taking into account the fact that she had always loved animals and nature, Gaynor made the decision to major in the department out of which the class was taught: Ecology, Evolution and Environmental Biology.
“Another deciding factor,” said Gaynor, “was that I felt welcomed, supported and like I belonged in the tight-knit Ecology, Evolution and Environmental Biology department. I actually came close to being a physics major in my first year, but I didn’t like the large class sizes, the competitive environment, and the hyper masculine culture of the field.”
Exploration remained key to Gaynor’s process.
She continued taking more classes that were slightly outside the zone of her knowledge — such as primatology — leading to her second major in biological anthropology. She also started testing out research opportunities by volunteering in a primate cognition lab, and studying abroad to do archaeology in Ecuador and field biology in Brazil. From these experiences, Gaynor realized that she was really drawn to fieldwork, leading her to travel to Kenya to complete her undergraduate thesis on vigilance behavior in blue monkeys under the supervision of Dr. Marina Cords.
Her undergraduate thesis research was Gaynor’s first taste of independent research: a project that she had designed and executed from start to finish. She was hooked. “I knew that was it. That’s what I wanted to do,” she said.
“After I graduated I went back to that field site in Western Kenya,worked for the professor who was my advisor in undergrad, and managed the research project for a year.”
Gaynor began thinking about graduate school. But before she applied, she wanted more experience as a researcher. She decided to broaden her skill set by working in Dr. Dustin Rubenstein’s lab investigating behavioral ecology (kin selection and social behavior) and genetics in snapping shrimp and starlings.
Her years as a researcher hugely informed Gaynor’s PhD project. While in Kenya, she observed monkeys feeding in trash pits, living in fragmented plantations, and eating the mud from the exterior of houses. In other words, these monkeys were living in anthropogenic contexts that were different from the context in which they had evolved.
“For my PhD, I decided to bring together my interest in animal behavior with my questions about the growing role of humans in the environment, ultimately hoping I could positively influence conservation outcomes,” said Gaynor.
Through continued fieldwork, Gaynor investigated the effects of human disturbance, especially hunting, on wildlife in Mozambique’s Gorongosa National Park. She observed that in some ecosystems, a “landscape of fear” emerged, in which animals respond to human disturbances by changing their behavior by, for example, becoming more nocturnal.
The research had informative results for conservation efforts, so Gaynor was motivated to remain in the same discipline and contribute further knowledge. For her postdoc, she wanted to bolster her data science skills after years of building up expertise in fieldwork, which led her to a three-year residence as a fellow at an environmental data synthesis center.
At UBC Gaynor will use her insights from across systems — from the study of animal behavior, field ecology and data science — to positively influence biodiversity and conservation.
We concluded by asking Gaynor a few more questions.
What tips would you give to students looking for research opportunities?
Answer: I got my first research position working in a primate cognition lab by cold-emailing the professor leading that lab. It was really scary! I understand the fear of reaching out to a professor and the vulnerability required to introduce yourself in an email, but ultimately I think you shouldn’t get discouraged. If one opportunity doesn’t work out, something else will.
Opportunities are not always going to be advertised far and wide, which is a real problem from an equity standpoint. But what this means for you, as a student, is that youoften have to ask your professors and advisers about what opportunities are available. Ask, and then be open to saying yes even if an opportunity isn’t exactly what you had envisioned.
I was open to a lot of experiences that weren’t part of any set plan but which I ultimately found really formative. My first experience with fieldwork came about after I saw a poster advertising a summer program in Brazil that involved research, which I applied and got into. I happened to mention to my academic advisor that I would be going to Brazil for a month out of my summer and that I didn’t know how to spend the rest of my break. My advisor informed me about a course he’d be teaching in Ecuador for the month after, and he encouraged me to apply and join his team as a volunteer, which I did. These international experiences were life-changing, and I wouldn’t have found them if I hadn’t kept my eyes open and asked questions.
Is there a benefit of taking classes outside of your main area of interest? Did you have difficult experiences taking classes you didn’t like?
Answer: There were definitely some classes that I was required to take that I was not enthused about. But, taking classes is all about exploring and defining your interests. You might be surprised like I was. I remember having to take a course in earth and atmospheric sciences, which didn’t seem to apply to my interests as an ecologist, but it ended up being one of my favorite classes because it increased my literacy around climate change, which is of course very relevant to modern ecology.
Not all my classes turned out to be interesting, though. There were aspects of my journey that were hard. There were aspects that were not fun. There were classes that I took that I really didn’t like. In those difficult moments, it can be hard not to take it all as a sign that you should quit.
But struggling in the learning and practice of science does not necessarily mean that it’s not right for you. Sometimes it can be a question of the learning environment. I’m trying to create more inclusive spaces where students can see themselves and feel welcome, and I encourage students to seek those spaces before leaving science.
What is a commonly held belief about science that you want to dispel?
Answer: Science does not necessarily have to happen in controlled settings, i.e. in the lab with microscopes and pipettes. The laboratory is a great way to do science for a lot of people. My method is with my hiking boots on, a pair of binoculars in hand, and a clipboard. Science is about coming up with an idea and finding a way to test it, and this can include other ways of knowing that are currently outside our traditional Western scientific framework.
Finally, I always like to share messages of hope around biodiversity. We are in a giant environmental crisis, but we don’t often get to hear about the successes of conservation efforts. There are a huge amount of people that are dedicating much of their time and energy towards restoring landscapes. And I know that there are students who are waiting to apply their different skills to make things better. I hope I can encourage and help those students.
We’re excited about the opportunity to collaborate with Gaynor’s research program. You can find more information about her ongoing work by checking out:
Congratulation to Michelle Tseng and her collaborators on being one of the recipients of the 2022 Campus as a Living Lab Fund Competition, which this year partnered with Wellbeing to expand the opportunities provided through the competition and support more projects advancing new ideas and innovations in Wellbeing, for their project Campus Trees, Microbes and Insects (CTMAIN): A database for understanding how tree traits mitigate climate change and enhance biodiversity
Dr. Celeste Leander, Professor of Teaching in the Departments of Botany and Zoology, has received a 2023 West Coast Teaching Excellence Award. The honour, awarded to top post secondary teachers in British Columbia and the Yukon by the BC Teaching and Learning Council (BCTLC), recognizes Dr. Leander’s deep commitment to student-centred teaching.
“Celeste makes brave choices in implementing new approaches in the service of supporting students,” said Dr. Simon Bates, UBC’s Vice-Provost and Associate Vice-President, Teaching and Learning, pro tem. “She is a role model for students to venture into uncertain territory, take risks, and to view the endeavour, regardless of the results, as a learning opportunity.”
A UBC Killam Teaching prize recipient, Dr. Leander was a pioneer in designing and implementing a flipped classroom in UBC’s first-year biology program. She was also the first in her field to establish and administer cooperative exams. Distinct from two-staged exams, which require students to first turn in their exams individually and then collaborate with their peers to answer the same questions again, cooperative exams encourage collaboration from the get-go. Dr. Leander designed questions that would lend themselves to teamwork through lively discussions, and in some cases, video-graphed answers.
“Celeste makes brave choices in implementing new approaches in the service of supporting students.” Dr. Leander was also the first to adopt “ungrading” in an upper-year biology course. Ungrading shifts the power dynamic between students and teachers by allowing students to submit a suggested letter grade for themselves along with documentation critically evaluating their own work. Her success in implementing ungrading has resulted in a request to deliver the same assessment practice in a large first-year biology course as well.
“We’re delighted to be shining a light on great teaching in B.C. and the Yukon,” said Maxwell Stevenson, chair of the 2023 BCTLC awards adjudication committee. “We received many outstanding nominations for individuals who are all doing inspiring work in our sector. Even though we could only make five awards, we were grateful for the opportunity to learn from each of the nominees.”
Dr. Leander has authored several teaching case studies in the National Science Teaching Association database and has been praised for her kindness and dedication to prioritizing student learning. She has also been credited for creating a welcoming and stress-free classroom environment, with students responding particularly positively to her adoption of the ungrading assessment practice.
The delicate stalk and pretty white flowers of Triantha occidentalis may seem like the perfect place to perch if you’re an insect, but get trapped in its sticky hairs and it will suck the nutrients from your dead corpse.
That’s the surprising new finding by University of British Columbia and University of Wisconsin-Madison researchers, detailed in PNAS.
Triantha – a species of false asphodel – is the first new carnivorous plant to be identified by botanists in 20 years. It is notable for the unusual way it traps prey with sticky hairs on its flowering stem.
“Carnivorous plants have fascinated people since the Victorian era because they turn the usual order of things on its head: this is a plant eating animals,” said co-author Dr. Sean Graham, a professor in the department of botany at UBC. “We’re thrilled to have identified one growing right here in our own backyard on the west coast.”
The plant grows in nutrient-poor, boggy but bright areas on the west coast of North America, from California to Alaska. For the study, the researchers investigated specimens growing on Cypress Mountain in North Vancouver, British Columbia.
“What’s particularly unique about this carnivorous plant is that it traps insects near its insect-pollinated flowers,” said lead author Dr. Qianshi Lin, a PhD student at UBC botany at the time of the study. “On the surface, this seems like a conflict between carnivory and pollination because you don’t want to kill the insects that are helping you reproduce.”
“We believe that Triantha is able to balance carnivory with pollination because its glandular hairs are not very sticky and can only trap midges and other small insects, so that the much larger and stronger bees and butterflies that act as its pollinators are not captured,” said co-author Dr. Tom Givnish, a professor in the department of botany at the University of Wisconsin-Madison.
The research builds on previous work in Dr. Graham’s lab, which found that Triantha lacked a particular gene that is often missing in other carnivorous plants.
In order to investigate if the plant was indeed partial to snacking on insects, Dr. Lin attached fruit flies labelled with nitrogen-15 isotopes to its flowering stem. The label acted like a tracking device, allowing Dr. Lin to trace changes in nitrogen uptake by the plant.The research builds on previous work in Dr. Graham’s lab, which found that Triantha lacked a particular gene that is often missing in other carnivorous plants.
He then compared the results with those from similar experiments on other species that grow in the same area, including a recognized carnivorous plant (a sundew) and several non-carnivorous plants as controls.
Isotopic analysis showed significant uptake of nitrogen by Triantha, which obtained more than half its nitrogen from prey –comparable to sundews in the same habitat, and other carnivorous plants elsewhere.
The study also found that the sticky hairs on the Triantha flower stalk produce phosphatase, a digestive enzyme used by many carnivorous plants to obtain phosphorous from prey.
The proximity of Triantha to major urban centres in western Canada and the Pacific coast in the United States suggests that other carnivorous plants – and many other ecological surprises – remain to be discovered, even in well-studied ecosystems.
But if you’re tempted to recreate the film, Little Shop of Horrors, or bring Triantha home to deal with pesky summer fruit flies, the researchers warn the plant doesn’t do well outside of its natural environment and advise admiring its quirks from a distance.
Figure 1: Flower of Triantha occidentalis in a bog at Cypress Provincial Park, British Columbia. Photo: Danilo Lima.
Figure 2: Triantha occidentalis traps insects. Photo: Dr. Qianshi Lin
Figure 3: The bog at Cypress Provincial Park. Photo: Dr. Qianshi Lin
Figure 4: The first new carnivorous plant identified by botanists in 20 years. Photo: Danilo Lima
Massive blocks of genes—inherited together ‘plug and play’ style—may play a larger role in evolutionary adaption than previously thought, according to new research in Nature.
Biologists identified 37 of these so-called ‘supergenes’ in wild sunflower populations, and found they govern the modular transfer of a large range of traits important for adaptation to local habitats. Those include seed size, timing of flowering, as well as the ability to withstand environmental stresses such as drought or limited nutrient availability, among many others.
“We were quite surprised,” says University of British Columbia (UBC) geneticist Marco Todesco. “Cases in which individual supergenes controlled adaptive traits had been reported before, but it wasn’t clear if they were the rule or just a small number of odd exceptions. What found is that supergenes have a pervasive role in adaptation, and can be truly massive.”
Large blocks of ‘plug and play’ genes play a super-sized role in adaption–and may help fill lingering gaps in Darwin’s theories.
The largest of the supergenes identified in the study is comprised of more than 100 million base pairs (larger than many human chromosomes) and 1,819 genes.
The study could help resolve a question left unanswered by Darwin’s theory of natural selection—namely, how populations of organisms that live side-by-side and mate with each other are still able to adapt unique traits and diverge into separate species.
“Initially, evolutionary biologists believed that geographic isolation between populations was required for them to differentiate into ecological races or separate species,” says UBC evolutionary biologist Loren Rieseberg. “But recent research shows that populations that exist side by side can, and do, differentiate.”
“The traits that govern such differentiation often appear to be inherited together as supergenes despite genetic exchange with non-adapted populations that are nearby. In many cases, plants are able to adapt to a new environment by borrowing a supergene or two from a related species that is already adapted.”
Examples of habitats in which supergenes played a major role in sunflower species adaption include the Texas coastal plain, sand dunes, and coastal barrier islands of the Gulf of Mexico. In the latter case, a 30 million base pair-long supergene controls a difference in flowering time of more than two and half months between sunflowers adapted to Texas’ barrier islands and coastal plains. The early-flowering version of the supergene found in the barrier island populations came originally from the common sunflower.
In some instances, the donor species for the supergene might be extinct. “What we think could have happened is that a species arrives in a new habitat, ‘steals’ adaptive supergenes from a local related species, and then replaces that species,” says Todesco. “We could call this a ‘ghost supergene’, the lingering contribution of a species that no longer exist.”
Because of their diversity and ability to adapt also to inhospitable habitats, wild sunflowers have become a model system for evolutionary studies.
“Genome BC has been investing in this work since 2009,” says Lisey Mascarenhas, director, Agrifood and Natural Resources at Genome BC. “A convergence of vision, strategic investments, and scientific leadership has helped propel innovations in sunflower genomics research that will have significant implications for food security and continue to attract global investment to BC.”
The researchers sequenced the genomes of more than 1,500 plants from three wild sunflower species: the common sunflower (Helianthus annuus), prairie sunflower (Helianthus petiolaris), and silverleaf sunflower (Helianthus argophyllus). They then looked at associations between genetic variants and more than 80 traits that they monitored throughout the plants’ growth, as well as with the soil and climate of their populations of origin. The result is the largest and most comprehensive demonstration to date that structural variants–rearrangements of chromosome structure that are largely responsible for creating the supergenes in the first place–play a fundamental and widespread role in adaptation and speciation.
In addition to the supergenes, the study also identified numerous independent genes that appear to confer resistance to the environmental stresses wild sunflowers face, including drought, heat and low nutrient stress. These independent genes will be invaluable to sunflower breeders as they develop cultivars that can tolerate the more extreme growing conditions predicted under future climate change. From an agricultural standpoint, they offer more flexibility than the supergenes.
“Because they work as a package, introducing a supergene into a cultivated sunflower would mean carrying over both the beneficial and detrimental traits associated with it,’ says Todesco. “While supergenes contain several genes that could be beneficial in an agricultural setting, they also contain hundreds of other genes, some of which might not be so beneficial in a crop. For example, by reducing yield or modifying the oil content of seeds.”
Related Links:
Figure 1: Supergenes play an outsized role in maintaining wild sunflowers’ extensive variation. Credit: Marco Todesco, University of British Columbia.
Figure 2: Silverleaf sunflowers found on coastal barrier islands (left) flower much earlier than plants growing in nearby plains (right). Brook T. Moyers, University of British Columbia.
Figure 3: Supergenes help the prairie sunflower adapt to the harsh environment of sand dunes. Credit: Nolan C. Kane, University of Colorado Boulder.
Article by: Miranda Meents (Lacey Samuels lab)
2015 is somewhat of an anniversary in cell biology. 350 years ago, Robert Hooke published the first description of what he called a cell. But it wasn’t until the mid-19th century that we started to look inside cells. Then, in 1898, the Italian scientist Camillo Golgi first described, and gave his name to, a cell structure that is the hero of this story, the Golgi Apparatus. We now know that the Golgi is a stack of flattened compartments like a stack of pita bread. Inside the pita pockets hundreds of chemical reactions occur as the Golgi makes, modifies and packages materials for the cell. Every animal, plant, and fungal cell has a Golgi. In plants, the Golgi is where many key components of the plant cell wall are produced. In humans, defects in Golgi structure or function have been associated with Alzheimer’s and Parkinson’s disease, as well as a growing list of less well-known disorders including neurological, skeletal, developmental, muscular, and more. But, despite close to 120 years of research, we still don’t really understand the basic mechanism behind Golgi function, and this is the question that my research is helping to address.
If we cut through the middle of the Golgi, in each pocket we would see a mix of what we call residents and cargo. The residents live in the Golgi; they are the workers that make and modify the cargo. The cargo is what is being modified and packaged before it leaves the Golgi to move somewhere else in the cell. It might help to think of the Golgi as a can of mixed nuts. Some of the nuts are like Golgi cargo, say pecans, and we need to quickly pull them out so they can get to where they need to go. Other nuts are like residents, cashews for example. To carry out their function they have to stay in the mix. But how do we quickly and efficiently remove the pecans while leaving the cashews behind? This question has been extremely difficult to answer because the Golgi is very small, very active, and very efficient. The system I am using, however, allows me to work around these problems and determine how the Golgi functions.
I am mapping the location of residents and cargo within the Golgi. To do this, I have created fluorescently tagged residents that allow me to label the Golgi in plant cells. I can then find these residents and their cargo within the Golgi in high resolution images taken with an electron microscope. I can then compare the location of the residents and cargo and use the information to build a hypothesis about how the Golgi is able to sort through all of its contents, as well as it does. Ultimately, this research could influence an astonishing breadth of disciplines, from medicine to biofuels to beer, simply because the Golgi is such a key player in how all types of organisms function.
Article by Jin-Jin Hu (Turkington & Zhou lab)
We have been collaborating with colleagues in Yunnan Province, China to determine if stomatal frequency in Quercus guajavifolia could be used to estimate palaeo-CO2 levels. The work was spear-headed by Jin-Jin Hu and Zhe-Kun Zhou at the Key Laboratory for Plant Diversity and Biogeography of East Asia, Kunming, and at the Xishuangbanna Tropical Botanical Garden. Palaeoatmospheric CO2 concentrationestimates provide important palaeoenvironmental information in geological time and also a baseline reference for understanding future climatic change. Atmospheric CO2 concentrationhas been hypothesized to be a primary determinant of global climate change; periods of low atmospheric CO2 concentrations witnessed major glaciations, whereas those with higher CO2 concentrations had warmer conditions. This CO2-temperature relationship is conspicuous during the Quaternary. This relationship has also been confirmed for other time periods: for example, the Palaeocene-Eocene thermal maximum (PETM) was a brief but intense interval of global warming associated with elevated atmospheric CO2 concentration. Additionally, CO2 levels play a crucial role in affecting ecological and physiological characters of plants. Many previous attempts have been made to estimate palaeoatmospheric CO2 concentration because of the important role of atmospheric CO2 in the Earth system.
In our study we made these estimates of palaeo-CO2 levels as follows. First, we identified leaves of fossil species and then identified a close living relative of the fossil species. In our study we used Quercus preguyavaefolia fossils from two late Pliocene floras in southwestern China, and Quercus guajavifolia (Fagaceae), an extant dominant element of sclerophyllous forests (evergreen trees and shrubs typically with small, hard, thick, and leathery leaves) along the steep altitudinal gradients in the Himalayas, Qinghai-Tibet Plateau and Hengduan Mountains. Second, we collected leaves of Q. guajavifolia collected from17 field sites at altitudes ranging from 2493-4497 m, mostly from the border regions between Yunnan and Sichuan Provinces. A range in altitude is critical because the change in elevation also represents a gradient in pCO2 (CO2 partial pressure). At all locations we collected both sun leaves (collected from outer branches) and shade leaves (from within and beneath canopies) because it is known that light intensity affects these two types of leaves differently and has an effect on the stomatal frequency. In addition we also examined herbarium samples of Q. guajavifolia collected during the time period 1926-1995. Just as a change in elevation provides a spatial change in pCO2, so the years 1926-1995 provide a temporal change in pCO2 levels. Third, we measured the stomatal frequency of all leaves – fossil leaves, living collection and herbarium samples. Fourth, we determined how the stomatal frequency of Q. guajavifolia responded to decreasing pCO2 (increasing altitude) and used this to generate calibration curves of stomatal frequency versus atmospheric pCO2. However, the fossil leaves were identified as sun leaves so the calibration curve for CO2 estimation was prepared exclusively from sun leaves of Q. guajavifolia. The samples collected along the altitudinal gradient provided results consistent with results from the historical herbarium specimens. Finally, we used these calibration curves along with the stomatal counts from the Q. preguyavaefolia fossils to estimate palaeo-CO2 from two late Pliocene floras in southwestern China.
In most previous studies using over 170 plant species, researchers generally detected an inverse relationship between atmospheric CO2 partial pressure (pCO2) and leaf stomatal frequency i.e. stomatal frequency decreased as pCO2 increased has frequently been shown and this relationship has been widely used to estimate palaeo-CO2 levels. In contrast, we detected a positive correlation between pCO2 and stomatal frequency in Q. guajavifolia from both extant field collections and historical herbarium specimens. Applying these correlations to fossils, palaeo-CO2 concentrations are estimated to be c. 180-240 ppm in the late Pliocene which is consistent with most other estimations.
To view the article click on this PDF.
Jin-Jin Hu; Yao-Wu Xing; Roy Turkington; Frederic M. B. Jacques; Tao Su; Yong-Jiang Huang; Zhe-Kun Zhou. A new positive relationship between pCO2 and stomatal frequency in Quercus guyavifolia (Fagaceae): a potential proxy for palaeo-CO2 levels. Annals of Botany 2015; doi: 10.1093/aob/mcv007
Caption: Comparisons of leaf morphology of extant Quercus guyavifolia and fossil Q. preguyavaefolia. (A, B) Branches of extant Q. guyavifolia. (C, D) Cleared leaves of extant Q. guyavifolia. (E, F) and (G, H) are leaf fossils from the Hunshuitang flora and the Qingfucun flora respectively. Scale bars = 1 cm.
Viruses and bacteria fall back to Earth via dust storms and precipitation. 2011 dust storm in the Sahara.
An astonishing number of viruses are circulating around the Earth’s atmosphere – and falling from it – according to new research from scientists in Canada, Spain and the U.S.
The study marks the first time scientists have quantified the viruses being swept up from the Earth’s surface into the free troposphere, that layer of atmosphere beyond Earth’s weather systems but below the stratosphere where jet airplanes fly. The viruses can be carried thousands of kilometres there before being deposited back onto the Earth’s surface.
“Every day, more than 800 million viruses are deposited per square metre above the planetary boundary layer—that’s 25 viruses for each person in Canada,” said University of British Columbia virologist Curtis Suttle (a professor in the Departments of Botany, EOAS, and Microbiology & Immunology, and the Institute for the Oceans & Fisheries), and one of the senior authors of a paper in the International Society for Microbial Ecology Journal that outlines the findings.
The findings may explain why genetically identical viruses are often found in very different environments around the globe.
“Roughly 20 years ago we began finding genetically similar viruses occurring in very different environments around the globe,” says Suttle. “This preponderance of long-residence viruses travelling the atmosphere likely explains why—it’s quite conceivable to have a virus swept up into the atmosphere on one continent and deposited on another.”
Bacteria and viruses are swept up in the atmosphere in small particles from soil-dust and sea spray.
Suttle and colleagues at the University of Granada and San Diego State University wanted to know how much of that material is carried up above the atmospheric boundary layer above 2,500 to 3,000 metres. At that altitude, particles are subject to long-range transport unlike particles lower in the atmosphere.
Using platform sites high in Spain’s Sierra Nevada Mountains, the researchers found billions of viruses and tens of millions of bacteria are being deposited per square metre per day. The deposition rates for viruses were nine to 461 times greater than the rates for bacteria.
“Bacteria and viruses are typically deposited back to Earth via rain events and Saharan dust intrusions. However, the rain was less efficient removing viruses from the atmosphere,” said author and microbial ecologist Isabel Reche from the University of Granada.
The researchers also found the majority of the viruses carried signatures indicating they had been swept up into the air from sea spray. The viruses tend to hitch rides on smaller, lighter, organic particles suspended in air and gas, meaning they can stay aloft in the atmosphere longer.
Two newly discovered organisms point to the existence of an ancient organism that resembled a tiny version of the lumbering, human-eating science fiction plants known as ‘triffids,’ according to research in Nature.
The microscopic protists Rhodelphis limneticus and Rhodelphis marinus are genetically ‘sisters’ to red algae, but couldn’t be more different. Red algae are fleshy, large organisms with a simple genome that perform photosynthesis, just like plants. Rhodelphis are single-cell predators with a large, complex genome.
The two protists have a chloroplast, though it is not photosynthetic anymore, pointing to their close ties with plants in the distant past. They also have flagella, a whip-like structure which allows them to move and hunt for their dinner.
“Rhodelphis shows that there was a period of time when the ancestors of plants and algae probably absorbed sunlight to generate energy, while also swimming around eating things,” says University of British Columbia (UBC) biologist Patrick Keeling, the senior researcher leading the study.
If we think of life as a big family, with algae and Rhodelphis as sisters, their ancient mother was more like a triffid than your standard plant. Triffids are the tall, mobile, carnivorous plants featured in John Wyndham’s 1951 novel The Day of the Triffids.
This surprising evolutionary twist emphasizes the need for robust sampling in order to reconstruct a more complete picture of life.
“Most people don’t look twice at organisms like this under a microscope, and getting them into culture may be hard work but it’s the only way to really see the true diversity of life,” says Denis Tikhonenkov, the microbiologist who first captured the tiny predators and splits his time between UBC and the Russian Academy of Science.
“There are gems in nature we haven’t found, and sadly the importance of ‘old-fashioned’ exploration is being forgotten,” Keeling says. “These new lineages are a great example — making us realize we were previously seeing things backwards and now we recognize plants had ancestors we couldn’t have imagined.”
Keeling and Tikhonenkov worked on the project with the outstanding Russian Academy of Sciences protistologist Alexander Mylnikov, who died after a long illness shortly before the paper came to press.
“Rhodelphis shows that there was a period of time when the ancestors of plants and algae probably absorbed sunlight to generate energy, while also swimming around eating things.”
Non-photosynthetic predators are sister to red algae
Silvia Moreno-Garcia
silvia.moreno-garcia@science.ubc.ca
604.827.5001
An international team of researchers have completed a massive effort to sequence genes from more than 1,100 plant species—an undertaking that saw UBC botanists collect rare mosses from remote corners of BC, and travel to the South Pacific to collect parasitic plants.
“One of the crucial samples we wanted to include was a parasitic conifer shrub, Parasitaxus, which lives by feeding off fungi and another conifer,” says UBC botanist Sean Graham, who coordinated collection of 300 species for the One Thousand Plant Transcriptomes Initiative (1KP). “Instead of using sunlight to fix energy through photosynthesis, it behaves like a plant vampire.”
It was a particularly challenging sample to collect, says Graham. “Local colleague Adrien Wulff collected it from the field in New Caledonia and we set up an international supply chain to bring our frozen sample from the South Pacific, through the US, and on to Shenzhen, China, where the plant transcriptomes were sequenced. Our vampire plant was a very well-seasoned traveller!”
Our study confirms that the ‘impossible’ moss defines one of the two earliest branches of moss evolution.
Many of key plant species belong to moss families and were found closer to home, including the UBC campus. But collecting British Columbia’s so-called ‘impossible moss’ (Takakia lepidozioides) involved a boat trip and some wading up Jervis Inlet, to reach the southern-most population of this BC moss.
“It seems a bit mad to do an expedition to get this one moss, but it was a key plant to include. Takakia is a kind of living fossil that blends features with other ancient branches of plant life,” says Graham. “Our study confirms that the impossible moss defines one of the two earliest branches of moss evolution.”
The findings, published today in Nature, reveal the timing of whole genome duplications and the origins, expansions and contractions of gene families contributing to fundamental genetic innovations enabling the evolution of green algae, mosses, ferns, conifer trees, flowering plants and all other green plant lineages. The history of how and when plants secured the ability to grow tall, and make seeds, flowers and fruits provides a framework for understanding plant diversity around the planet including annual crops and long-lived forest tree species.
“In the tree of life, everything is interrelated,” said Gane Ka-Shu Wong, lead investigator and professor in the University of Alberta’s Department of Biological Sciences. “And if we want to understand how the tree of life works, we need to examine the relationships between species. That’s where genetic sequencing comes in.”
UBC researchers provided 60 plant samples to the study from across British Columbia, including 30 from UBC Botanical Garden.
“UBC Botanical Garden has fantastic collections of wild plants from around the world, including species from the from southern hemisphere,” says Graham. “This study underscores the importance of maintaining strong research collections around the globe, not only for scientific purposes, but as a way to conserve biodiversity.”
Students also helped out by growing fern species as part of undergraduate labs in the UBC biology program.
“Our inferred relationships among living plant species inform us that over the billion years since an ancestral green algal species split into two separate evolutionary lineages, one including flowering plants, land plants and related algal groups and the other comprising a diverse array of green algae, plant evolution has been punctuated with innovations and periods of rapid diversification,” said James Leebens-Mack, professor of plant biology in the University of Georgia Franklin College of Arts and Sciences and co-corresponding author on the study.
“In order to link what we know about gene and genome evolution to a growing understanding of gene function in flowering plant, moss and algal organisms, we needed to generate new data to better reflect gene diversity among all green plant lineages.”
The study inspired a community effort to gather and sequence diverse plant lineages derived from terrestrial and aquatic habitats on a global scale. Over 100 taxonomic specialists contributed material from field and living collections that from around the world, including Canadian collections from the University of British Columbia Botanical Garden and the University of Alberta. Links to some of the UBC living plants used in the study are posted at the UBC Botanical Garden.
By sequencing and analyzing genes from a broad sampling of plant species, researchers are better able to reconstruct gene content in the ancestors of all crops and model plant species, and gain a more complete picture of the gene and genome duplications that enabled evolutionary innovations.
Cannabis flowers with the most mushroom-shaped hairs pack the biggest cannabinoid and fragrance punch, according to new research from the University of British Columbia.
While the cannabis leaf is iconic, it’s the chemicals produced by the tiny, frostlike hairs on cannabis flowers that give the plant its psychoactive and medicinal properties and distinctive smell.
In a study published in The Plant Journal, UBC researchers have revealed the unique structures and chemical outputs of the different types of hairs, or glandular trichomes, for the first time.
“Despite its high economic value, our understanding of the biology of the cannabis plant is still in its infancy due to restricted legal access.”
Their findings confirm what many cannabis connoisseurs have long suspected: that the largest, mushroom-shaped stalked glandular trichomes are the richest source of THC- and CBD-forming metabolites and fragrance-giving terpenes.
“Despite its high economic value, our understanding of the biology of the cannabis plant is still in its infancy due to restricted legal access,” said co-lead author Teagen Quilichini, a postdoctoral fellow at UBC botany and Anandia Laboratories Inc. “Trichomes are the biochemical factories of the cannabis plant and this study is the foundation for understanding how they make and store their valuable products.”
Previous research had identified three types of glandular trichomes based on their appearance – bulbous, sessile and stalked – but their relative contributions to the chemical production of cannabis flowers were unknown.
For this study, the UBC researchers used a combination of advanced microscope techniques and chemical profiling to examine the internal structures and development of individual trichomes in a fast-flowering hemp variety of Cannabis sativa called ‘Finola.’
They found that under ultraviolet light, the stalked trichomes emitted a bright blue colour and contained a large, distinctive pie-shaped disc of cells. The smaller sessile trichomes, which do not have a stalk, emitted a red colour, had smaller secretory discs, and produced fewer fragrant terpenes.
“We saw that stalked glandular trichomes have expanded “cellular factories” to make more cannabinoids and fragrant terpenes,” said co-lead author Sam Livingston, a PhD candidate at UBC botany. “We also found that they grow from sessile-like precursors and undergo a dramatic shift during development that can be visualized using new microscopy tools.
As a result, Livingston explains, UV light could be used to monitor trichome maturity on flowers and inform optimal harvest times.
The researchers also conducted a gene expression analysis to investigate how instructions in trichome DNA are converted into the plant’s biochemical products. They found that the stalked trichomes in Finola were strongly geared towards making cannabidiolic acid (CBDA) and terpenes.
“We found a treasure trove of genes that support the production of cannabinoids and terpenes,” said principal investigator Anne Lacey Samuels, a botany professor at UBC. “With further investigation, this could be used to produce desirable traits like more productive marijuana strains or strains with specific cannabinoid and terpene profiles using molecular genetics and conventional breeding techniques.”
Next, the researchers will investigate how trichomes export and store the metabolites they produce.
“Trichomes store the metabolites in their cell walls,” said Livingston. “And what’s really astounding is that such high levels of product should be toxic to the cells, so we want to understand how they manage this.”
The research was funded by the Natural Science and Engineering Research Council of Canada (NSERC) and a MITACS Elevate postdoctoral fellowship, in partnership with Anandia Laboratories.
For most of us, microbes mean only one thing: disease. Disease-causing microbes are actually the extreme minority of the most abundant form of life on Earth. But because of their immediate and direct importance to our health, they are much better studied than the rest of the microbial world. Still, new discoveries about the basic biology and evolution of some of the most infamous pathogens can throw us a curveball.
My lab works on the evolution of apicomplexans, a diverse group of parasites that can only survive by infecting and living inside animal cells. For every animal scientists have studied, there’s an apicomplexan that infects it. A number of common and serious human pathogens, most famously the parasite that causes malaria, Plasmodium, are apicomplexans.
Even after 100 years of work, malaria researchers overlooked one bizarre feature of the parasite, a cellular compartment called a plastid, better known as a chloroplast. The plastid is the part of plant and algal cells where photosynthesis takes place. They’re highly-simplified relicts of photosynthetic bacteria that took up residence in an ancient ancestor of plants and algae. Today, plastids have become genetically integrated with their hosts and are an essential part of plant and algae cells.
But how did a parasite like malaria, which lives in the blood of humans, come to possess a plastid? They certainly don’t do photosynthesis, so why do they have plastids? To an evolutionary biologist interested in endosymbiosis — cells living within other cells — it’s a hard question to resist. But it’s also a challenging question to study. Apicomplexan plastids are more than a little weird, and both their genomes and structures are severely simplified from their original form, making them hard to compare to those of plants and algae.
The answer to this question came out of the blue, literally. It was the exploration of coral reefs that provided clues to the origin of apicomplexan plastids. Coral reefs are built thanks to an intimate endosymbiotic relationship between the coral animals and an alga called Symbiodinium. Then Australian researchers discovered some corals contain another symbiont, an apicomplexan whose plastid was still photosynthetic. The discovery took my research in an entirely new direction. The new apicomplexan suggested not only an evolutionary link between the parasites and their photosynthetic ancestors but also to coral, one of the most ancient animal lineages. Perhaps apicomplexans began an association with animals in a different context — as beneficial symbionts instead of parasites.
To follow this lead, however, we had to think beyond the initial evolutionary questions about the origin of the parasites. We had to start to use the tools and methods of environmental microbiology to discover more about the diversity, biology and ecology of apicomplexans in coral. We also moved our experiments from the lab into the field. Luckily these major leaps were not so hard because we were part of a microbial biodiversity network that included experts in coral microbiology. It became the start of a long collaboration with the Caribbean Research and Management of Biodiversity research station.
In 2013, we packed up our microscopes and scuba gear and flew to one of the southernmost islands of the Caribbean, Curaçao. Thus began the first of four expeditions where we examined the microbes associated with corals, including the enigmatic apicomplexans.
The leap into environmental research paid off, and the expeditions successfully led to our discovery of a widespread and diverse lineage of apicomplexans living inside corals and related animals like anemones, black corals and sea fans. These new coral-associates are not photosynthetic, but surprisingly they still had the primary pigment that collects light — chlorophyll. It was another unexpected glimpse into the transition from symbiont to parasite.
The environmental research gamble paid even more unanticipated dividends. Taking a group of computer-bound molecular biologists, genomics students and postdocs into the field not only heightened their motivation but put our work into a different context. Most members of my lab are now certified scuba divers. For me, this is one of the more rewarding aspects of the project.
None of us became biologists to sit at a computer and respond to emails, but somewhere in the last 40 years, biology has lost some of the sense of adventure and exploration that made Jacque Cousteau and National Geographic staples of what little TV I watched as a kid. But exploration is the backbone of our work. Even today we know so little about the microbial world that surrounds us that it can be difficult to even know what questions we should be asking.
To ask the right questions, you sometimes have to start by looking around.
By 2019 Wall Scholar Curtis Suttle, a Professor and Fellow of the Royal Society of Canada at the University of British Columbia, where he shares an office with billions of viruses.
The novel coronavirus, SARS-CoV-2, is just the latest in what seems to be a never-ending war against the spread of viral diseases; Measles, Influenza, HIV, Ebola, Zika, SARS. It is estimated that the Spanish flu killed 50 to 100 million people worldwide, and that smallpox killed more than 20 million people in North America alone, so it is no wonder that public health authorities are on edge about the emerging viral disease caused by the novel coronavirus. Indeed, the word “virus” is rarely mentioned in good news stories in the media. Thus, it is surprising that we wouldn’t exist and could not survive without them.
Viruses are tiny. If an average virus was the size of a pinhead, and an average height American was scaled accordingly, the person would be 150 km tall. Viruses are unlike other forms of life, and in fact, are often not considered to be alive, at all. They have no metabolism on their own, and no capacity to reproduce unless they infect a suitable host – viruses typically infect a specific host, analogous to a key opening a lock. It is this high host specificity that endows viruses with their critical role in maintaining life.
Viruses are everywhere. We are swathed by the hundreds of millions of viruses that fall from the atmosphere on each square foot of Earth, every day. In a tablespoon of ocean or lake water there are more viruses than people in North America, and most of us will swallow a billion viruses every time we swim in a lake or the ocean. If the viruses in the ocean were stretched end-to-end they would span 10 million light years, or farther than the nearest 60 galaxies. We are inundated by viruses that don’t make us sick.
But, clearly, viruses can also infect us; so, how is it that we would not exist without them? Our long and complex history with viruses dates back millions of years, and is written in the 8% of our DNA that is viral in origin. Every time a virus infection occurs, there is a very small probability that a bit of its genetic material will be left behind. Even though such events are exceedingly rare, over millions of years and billions of infections, genetic material has been left behind many times. In fact, our genome contains about 100,000 remnants of genes originating from viruses.
These contributions by viruses to our genetic makeup have been crucial to our evolution. One example is the placenta; if it were not for a gene donated by a virus there would be no placenta, and mammals would not exist. Another, is a gene that encodes a protein that moves information between cells in the nervous system, and which appears to be critical to long-term memory. Without the intervention of viruses, we would not exist as a species.
We could also not survive if viruses did not carry out ecosystem functions on which we depend. For example, about 95 percent of the living material in the oceans, by weight, is microbial, and these microbes produce about half of the oxygen on Earth. Viruses are estimated to kill about 20% of these organisms, each day. This continuous mortality liberates the nutrients that sustain oxygen-breathing organisms.
Viruses are also crucial for maintaining biodiversity. Not only does much of the Earth’s genetic diversity reside in viruses, they also help maintain biodiversity by infecting the organisms that would otherwise outcompete all others. This process, termed “kill the winner”, occurs because viruses spread rapidly among hosts when their density is high; thus reducing their population, and freeing up resources so other species can grow. Indeed, outbreaks of influenza and other viral infections occur every fall and winter as children head back to school and we crowd together indoors.
Viruses have been sculpting life on our planet for a very long time. They existed billions of years before our first multicellular ancestors emerged. Yet, through evolutionary time, humans and all life have found a way to co-exist with the viruses that infect them.
Paradoxically, even though viruses continue to make us ill, humans would not exist without viruses, and our ongoing survival depends on them. The central role of viruses in our evolution and survival is something that needs to be acknowledged in the classroom. Viruses get a lot of bad press, but their integral role in our survival is something that scientists and creationists should be able to agree on, and is worth pondering the next time a rhinovirus gives you a runny nose.
Curtis Suttle is a Distinguished University Scholar at UBC, and a professor in the departments of Earth, Ocean and Atmospheric Sciences, Microbiology & Immunology, Botany, and the Institute for the Oceans and Fisheries.
Article by George Haughn
The primary plant cell wall of land plants is an essential cellular structure needed for support, cell-cell adhesion, signaling and interaction with both the biotic and abiotic environment. It is composed of a complex, dynamic extracellular matrix consisting of a network of carbohydrate polymers (cellulose, hemicellulose and, pectin) and structural proteins. Wall composition can vary dramatically both throughout the life of individual cells and between different cell types. Such variation is necessary given the need for growth and differences in the function of various cell types. Due to its structural complexity, cell-cell variation and the paucity of analytical tools for complex carbohydrates, scientific progress toward understanding the cell wall has been relatively modest leaving large gaps in our knowledge. We are attempting to fill some of these gaps by using molecular genetics as an approach and the Arabidopsis seed coat as a model system.
The Arabidopsis seed coat has a specialized epidermis containing a large quantity of mucilage between a volcano shaped secondary cell wall and the primary cell wall. Upon exposure to water, the mucilage expands, rupturing the outer primary walls and encapsulating the seed to aid in dispersal, protection and/or hydration (Figure 1).
Figure 1. Arabidopsis seed with extruded mucilage stained with a red pectin specific dye.
Like primary cell walls, seed mucilage is composed of cellulose, hemicellulose, pectin and protein. When extruded, mucilage forms dark-staining rays perpendicular to the seed surface. The rays are adherent to the seed. Since mucilage has all the major components of the primary cell wall, is produced by a single cell type, is available in large quantities, has a distinct structure and is not required for seed viability, it is well suited as a genetic model for studying cell wall biogenesis, function and regulation.
Cellulose in extruded mucilage is a component of the ray that extends many cell lengths beyond the surface of the seed (Figure 2). Using molecular genetics we have investigated its function and how it is made and packaged in the seed coat epidermal cells (Mendu et al., 2011, Plant Physiol. 157: 441–453; Griffiths et al., 2015, Plant Physiol. 168: 502-520).
Figure 2. Arabidopsis seed with extruded mucilage stained with a red cellulose specific dye.
Mucilage matrix components (pectin, hemicellulose) are made in seed coat epidermal cells and secreted to the apoplast forming a mucilage pocket between the cell membrane and the primary cell wall (Figure 3).
Figure 3. Developing seed coat showing an epidermal cell with volcano shaped cytoplasm and a mucilage pocket (purple) between the cytoplasm and the outer primary cell wall.
Similar to cellulose synthesis in other cell walls, the cellulose in seed mucilage is made by cellulose synthase (CESA) complexes in the membrane. CESA3 and CESA5 are CESA subunit polypeptides that are part of the complex in seed coat epidermal cells and are found in the membrane lining the mucilage pocket (Figure 4).
Figure 4. Seed coat epidermal cell showing fluorescent CESA5 in the membrane around the developing mucilage pocket.
These complexes move in a linear, unidirectional fashion around the cytoplasmic column of the cell, parallel with the surface of the seed presumably synthesizing cellulose that is coiled around the volcano shaped cytoplasmic column. This cellu-lose appears to unravel as the mucilage extrudes, forming a linear ray (Figure 5).
Figure 5. Diagram showing cellulose and pectin in unextruded and extruded mucilage of a seed coat epidermal cell.
Both the speed of extrusion and the adherence of the mucilage are dependent on this cellulose. The fact that normal pectin is also seems to be required for both processes (Griffiths et al., 2014, Plant Physiology, 165: 991-1004) suggests that these unique properties of mucilage require an interaction between the cellulose and pectin components of mucilage.
Article by Samuel Starko and Patrick Martone
What physical factors influence the morphological variation of plants? How do different morphologies coexist, if they perform similar ecological roles? Marine, non-vascular plants (seaweeds) live in some of the most physically stressful habitats on the planet: wave-swept rocky shores. Seaweeds are constantly bombarded with powerful waves that can rip them off the rocks, causing death and providing an effective source of selective pressure for organisms that live under these conditions. Branching (i.e. the splitting of a frond into two or more parts) has evolved repeatedly in many different seaweed lineages, as a mechanism of thallus elaboration, suggesting a common selective advantage to such a form; however, this morphological diversification may come at a cost.
In our recent paper (Starko, S., Claman,B.Z. and P.T. Martone. 2015. New Phytologist, 206 (1) 133–140) we explore selective pressures acting on the morphological evolution of seaweeds. We created artificial seaweeds that were identical in “photosynthetic” area, cut from latex, and modeled after real branched and unbranched plants to quantify biomechanical differences between these ubiquitous (at least among seaweeds) forms. We showed that branching, even in two dimensions, significantly increases drag on model seaweeds in flow, potentially increasing the risk of dislodgement under crashing waves. Branched forms experienced greater drag at all test velocities, even after accounting for the ability of the seaweeds to fold up in flow. These results suggest that branching may have significant biomechanical consequences. So how do branched and unbranched seaweeds survive side-by-side on wave-swept shores?
By conducting a broad-scale meta-analysis, we further showed that real branched seaweeds attach more firmly to the substratum than unbranched seaweeds of similar size. And this pattern held true over 4 orders of magnitude size, from small seaweeds the size of your hand to 30-meter long giant kelps. This likely allows branched seaweeds to tolerate these increased forces and live along-side weaker, more streamlined forms. Interestingly, this trend was found regardless of phylogeny and differences between branched and unbranched forms were demonstrated for both brown (Heterokontophyta, Phaeophyceae) and red (Rhodophyta) algal phyla, suggesting that the conclusions of this study are a result of a biophysical phenomenon and not an artifact of organism relatedness.
Our study begs questions about the advantages to branching. Given the “cost” of compensating for increased drag with increased attachment, it seems likely that branching must be advantageous in some other regard. Branching may allow seaweeds to better respond to their environment, as it can allow them to adopt wider range of shapes and possibly supply some species with a greater ability to fine-tune their own morphological structure. Additionally, loss of individual branches by wave action may be less devastating to plants than the loss of an entire unbranched blade. Finally, branching can be associated with an increased surface area to volume ratio, which may increase nutrient transfer and improve rates of net primary production. Given these advantages, our study may explain how branched and unbranched seaweeds coexist, and why both morphologies, not just one, are so abundant across the phylogenies of different seaweed lineages.
Figure caption.
(A) The unbranched macroalga, Mazzaella flaccida, is found in intertidal habitats predominantly in California.
(B) The dichotomously branched macroalga, Fucus distichous, is commonly found in intertidal habitats throughout the NE Pacific.
Article by Katy Hind, Sandra Lindstrom, and Patrick Martone
It is estimated that we have described only 10-20% of species diversity on Earth. In particular, floristic surveys using modern molecular techniques have revealed that we are vastly underestimating the number of seaweed species. This finding has implications for researchers using classical methods of identification and is likely complicating studies of ecology and physiology.
At the Hakai Institute, on Calvert Island along the central coast of British Columbia, Drs. Katy Hind, Sandra Lindstrom, and Patrick Martone are using an integrative approach that combines genetics, ecology, biogeography and historical DNA to accurately assess species diversity. Over the last 5 years, more than 220 species of seaweeds have been documented from this region, from microscopic filaments to large habitat-forming kelps. In particular, they have described over 38 species of calcifying red algae, called coralline algae, which play a key role in the ecology of marine ecosystems and have been used increasingly in studies of climate change, ocean acidification and historical sea ice coverage. Coralline algae dominate the ocean floor in rocky subtidal habitats and comprise a large portion of the kelp forest understory.
In a recent study Hind and Martone documented three new coralline algal species from Calvert Island, including one that they named after the Hakai Luxvbalis Conservancy region (Bossiella hakaiensis sp. nov.) where robust and abundant collections of this species were made. In addition, they confirmed using DNA from collections made over the last 100 years that at least three species in the genus Bossiella are widely distributed from Baja California to Alaska.
Hind and Martone plan to continue their research at the Hakai Institute and focus their efforts on the coralline algae that make up the understory of the kelp forest ecosystems. Kelp forests are highly productive ecosystems that act as nursery grounds for juvenile fish and food and habitat for many marine species. The Pacific sea otter, Enhydra lutris, in particularhas strong connections with kelp forest ecosystems as they forage on invertebrates (especially sea urchins) in this habitat. Where sea otters have been present, they have kept the sea urchin population in check and the kelp forests flourish. However, where the sea otters are absent (either due to migration or human interactions) the sea urchin populations have exploded and consumed much of the kelp, leaving only a ‘pavement’ of robust coralline algae in their wake. The species diversity of coralline algae that persist and flourish under such intense herbivory is completely unknown, but may be higher than previously thought.
Hind and Martone plan to study the relationship between kelp density and coralline algal diversity across a gradient of otter occupation in central British Columbia with sample sites ranging from established kelp forests to urchin ‘barrens’. Their study will uniquely incorporate the use of molecular tools to accurately identify coralline algal species. This research has broad implications for understanding ecosystem dynamics and for ecologists worldwide who are striving to understand the resilience of coralline-dominated communities.
Figure 1. Seaweed diversity in a rocky intertidal zone on Calvert Island, Hakai Institute.
Figure 2. Sandra Lindstrom and helpers conduct a seaweed survey at a long term monitoring site at the Hakai Institute, Calvert Island.
Figure 3. Calcifying red algae (coralline algae) are very abundant in the rocky intertidal zone on Calvert Island, BC.
Figure 4. Coralline algae are comprised of species having either upright, usually jointed fronds and prostrate, crustose forms. Over 38 species of coralline algae have been documented at the Hakai Institute.
Article by Tegan Haslam (Kunst Lab)
The transition of plants to life on land required many adaptations for survival in an environment so radically different from the sea. Perhaps the most challenging aspect of terrestrial life is the necessity of retaining water, which makes up more than 70% of most non-woody plant parts. Plants have many tools to address this challenge: stomatal pores that regulate gas exchange and water vapor loss, a complex vasculature for transporting water throughout the plant, and a waxy cuticle which serves as a barrier on all primary, aerial surfaces, preventing direct transpiration out of plant cells into the environment.
Our lab uses molecular genetics to study the synthesis and secretion of cuticular wax. Cuticular wax is a unique feature of land plants, as are the biochemical pathways and enzymes which make wax. However, the synthesis of cuticular wax builds off of fatty acid metabolism, which is ubiquitous in life. We are interested in the first steps in wax metabolism in which fatty acids are elongated to exceptional lengths suitable for conversion to waxes. These 28-34 carbon fatty acids and their derivatives make an excellent water barrier on the plant surface as they are very hydrophobic and are solid at room temperature. Given these physical properties, it is not surprising that the elongation of fatty acids to exceptional lengths requires tight regulation and biochemical machinery distinct from that used to make fatty acids for the synthesis of primary metabolites like membrane and storage lipids.
We are characterizing a new protein family called CER2-LIKEs. CER2 and its homologs modulate the chain length specificity of the core fatty acid elongation machinery, and enable it to produce the 28-36 carbon-long fatty acids required for wax biosynthesis. The core elongase is used for the production of all fatty acids, from 16-36 carbons, but CER2-LIKEs only appear to play a role in the elongation of fatty acids longer than 28 carbons. Accordingly, CER2-LIKEs are unique to cuticle-bearing land plants, and are a sub-group of the plant-specific enzyme family of BAHD acyltransferases. Despite homology to BAHDs, we found that mutagenesis of the putative acyltransferase catalytic site of CER2 does not compromise its function. Therefore, CER2 must have different biochemical activity from that described for the BAHD acyltransferase family.
We have developed a biochemical assay in yeast to observe and dissect the activity of CER2-LIKEs, and have demonstrated that a CER2-LIKE with the core elongation machinery is all that is necessary and sufficient to make exceptionally long wax precursors. Moreover, different CER2-LIKE proteins impart different specificities; for example, CER2 enables elongation to 30 carbons, and CER2-LIKE1 enables elongation to 34 carbons. These subtle changes in chain length have important consequences for the structure and physical properties of the cuticle.
The biochemical function of CER2-LIKEs remains unknown, but our studies suggest that these proteins do not have catalytic activity. Based on our yeast assay and protein-protein interaction data, our best hypothesis is that CER2-LIKEs function as allosteric modifiers of ubiquitous components of the elongase machinery called condensing enzymes. In the absence of CER2-LIKEs, condensing enzymes determine specificity of the elongase, so it fits that interaction between CER2-LIKEs and condensing enzymes could jointly establish unique specificity during wax production. To elucidate this process, we are carrying out protein domain swaps, further protein-protein interaction experiments, and tertiary structure modeling focused on CER2 and condensing enzymes.
While there are other organisms that can make fatty acids up to 36 carbons and longer, the pathway plants use to make cuticular waxes is unique, as are CER2-LIKEs and their apparent effects on condensing enzyme specificity. Determining how CER2-LIKEs function is an important step towards understanding the metabolic adaptations of plants to their dry, terrestrial environment.
Figure 1: Water beading off tepals of a lily, which is a consequence of repulsion between water and the hydrophobic cuticle.
Figure 2: Cuticular wax morphology can make plant surfaces appear whitish and dull. The stem on the left is from a wild-type Arabidopsis plant, while the glossy green stem in the middle is a cer2 mutant, which has compromised wax biosynthesis and altered wax crystal structure. The plant on the right is the cer2 mutant complemented with a CER2 transgene, demonstrating that the lesion in the CER2 gene is responsible for the wax-deficient phenotype of the cer2 mutant.
Article by Carl Douglas
Do plants have different sexes, meaning distinct male and female individual organisms within a species? The separation of male and female sexual function into different individuals is called “dioecy” and is common in eukaryotes, occurring in 94% of animals. Yes – this occurs in plants but in contrast to animals it is uncommon, occurring in only 6% of flowering plant species. A recent publication from the research groups of Quentin Cronk, Carl Douglas, and collaborators in the journal Molecular Ecology sheds new light on the evolution of dioecy, with practical spin-offs.
The evolution of dioecy is often accompanied by sex chromosome evolution, and theory suggests that sex-determining loci expand to whole sex chromosomes over time, which are structurally and genetically divergent. For example the mammalian Y chromosome evolved about 170 million years ago and is degenerate (contains very few genes) relative to the non-sex related (autosome) chromosomal pair from which it evolved, and human X and Y sex chromosomes are morphologically distinct and easy to tell apart.
Dioecy is thought to be advantageous by ensuring outcrossing and optimal allocation of reproductive resources, but if so, why is it rare in plants? When present, how and why has it evolved in plants? And, in dioecious plant species, what is the molecular mechanism that tells a male plant to make flowers with only male organs, and a female plant flowers with only female organs? So far, definitive answers to these questions are lacking.
Dioecy has evolved independently in many different flowering plant lineages, and is found in some well-known plants such as papaya, Cannabis, persimmons, asparagus and date palm. In most dioecious plants no discernable sex chromosomes are present, and sex-determining genetic systems are imbedded in areas of reduced recombination in chromosomes that are mostly autosomal (non-sex related).
Populus species (poplars, cottonwoods and aspens) are considered to offer an excellent opportunity to study the evolution of sex chromosomes. Populus and Salix (willows), sister genera in the family Salicaceae, are composed exclusively of dioecious species, suggesting a single origin of dioecy around 65 million years ago when the family arose. Confounding such studies, however, “boy” and “girl” poplar trees are impossible to tell apart (they lack any obvious sexual dimorphism) until they reach sexual maturity and begin to flower at age 5 to 8. Also poplars and willows lack sex chromosomes and the exact location of the poplar sex-determining region, as well the genetic basis for dioecy in this group, has remained unknown. This frustrates poplar breeders who can’t tell which trees they can mate for over 5 years of growth, and makes it difficult to control the sexes of poplar trees planted in plantations (e.g. females can produce huge amounts of undesirable cottony seeds and it may be desirable to restrict their numbers) or to study the mechanism of sex determination on poplars and the suspected ecological and physiological differences between the poplar sexes.
Four postdoctoral fellows/research associates working with Department of Botany professors Quentin Cronk and Carl Douglas, together with a number of other collaborators at UBC (in the Genome Canada/Genome BC-funded POPCAN project) and from other institutions, recently collaborated on major breakthrough study of plant sex in a paper published in Molecular Ecology (Geraldes et al., 2015). Using collections of black cottonwood, or poplar, (Populus trichocarpa) trees grown at UBC’s Totem Field, and of balsam poplar (P. balsamifera) trees grown at the Agriculture and Agri-Food Canada Agroforestry Development Centre, Indian Head SK as their study organisms, Drs. Armando Geraldes and Charles Hefer employed genomic and population genetic approaches to pinpoint the genetic position that determines sex in these tree species. Drs. Armando Geraldes and Arnaud Capron used this information to devise a simple but elegant DNA-based assay to accurately determine the sex of any tree of these and related species before they are old enough to flower, or during most of the year when they are not flowering. Dr. Natalia Kolosova coordinated the isolation of over a hundred poplar DNA samples that went into the analyses.
The new information from the UBC Department of Botany team locates the poplar sex-determining region to a single small genetic locus, near its previously suspected chromosomal position. This was accomplished by comparing the full genome sequences of 68 poplar individuals, generated by the POPCAN project, that had started to flower, and by identifying genetic differences in these genomes (Single Nucleotide Polymorphisms, SNPs) that strictly correlated with sex in the same trees. This work shows definitely that, like mammals, poplars use an XY system to determine sex (females are XX, males are XY), and that the region displays a hallmark of sex-determining loci: strongly suppressed recombination (to maintain the integrity of X and Y regions of homologous chromosome pairs that would otherwise be broken up by recombination over multiple generations). There were several surprises in the work – one being the apparent very small size of the poplar sex locus (about 100,000 DNA base pairs, comprising only 13 genes). This is very much smaller than predicted, since theory suggests that over evolutionary time, such loci should expand, eventually to encompass whole chromosomes – and poplars have had plenty of time. Surprisingly, the age of the poplar sex locus was dated at about 6 million years based on the molecular clock, much younger than the presumed origin of dioecy in the Salicaceae (65 million years), indicating either it must have either independently ‘arisen’ several times, or that it has undergone rapid evolution, or “turnover” as documented in many animals. Consistent with both possibilities, while the sex locus is conserved in other poplars and cottonwoods (eastern cottonwood from North America and black poplar from Europe), it is distinct from the sex determining loci recently described for aspen and a willow species, which are also in the Salicaceae.
These mysteries, and the questions of which gene or genes in the poplar sex-determining locus could specify sex after a 5 to 8 year juvenile period and how different combinations of X and Y alleles of this gene(s) in the sex locus lead to development of distinct male or female flowers, will be the subject of future research. In the meantime, researchers can now determine the sex of any poplar tree of any age from which a small amount of DNA can be isolated (e.g., from a small leaf sample – analogous to a human blood sample), opening the door to commercial applications and to new studies on the ecology and biology of sex in wild populations of poplar trees.
This article is featured as a ‘From the Cover’ paper, a Molecular Ecology section that contains papers of exceptional interest to a wide audience that address significant questions in ecology, evolution, behaviour or conservation.
http://onlinelibrary.wiley.com/doi/10.1111/mec.13126/epdf
Figure 1: UBC Totem Field black cottonwood trial, April 2014 – a mixture of male and female individuals used in the study of Geraldes et al. (2015). Photo, Carl Douglas
Figure 2: Poplar male inflorescence (catkin). Photo courtesy of Raju Soolanayakanahally
Article by Matthew Bayly (Angert Lab).
A current important area of research effort involves understanding how species’ geographic distributions and range limits will respond to climate change. But for most species of interest we lack basic data on how their fitness changes along climatic gradients. In many cases distribution data from atlases, herbarium specimens and vegetation surveys represent our best understanding of a species climatic niche (its range of tolerable conditions). Using these records we can then develop correlative relationships with key environmental variables and project these relationships into the future under different climate change scenarios.
This framework is widely used but a major challenge with this correlative approach is that we make the assumption that species’ distributions and their range limits are set entirely by their physiological limits. This assumption is routinely violated in wild populations. For example, in some cases areas beyond the range limit of a species might have high climatic suitability for growth and survival, but a species may fail to occupy these localities because of their inability to disperse seeds and reach these areas. Conversely, in some situations, a species may be present but only as a temporary resident (a sink populations) in a climatically unsuitable area.
Ideally we would like to know a population’s growth rate (the finite rate, lambda) at each site. We can estimate growth rates from large scale demographic surveys (birth and death statistics) of wild populations. Field transplants within, and beyond, a range limit are also invaluable as a means to test for dispersal limitation and experimentally validate the assumptions of our models. Unfortunately both broad scale demographic surveys and field transplant studies involve herculean effort, and in many cases are extremely difficult or impossible. This often drives us back to a reliance on data from atlases, herbarium specimens and vegetation surveys.
In the Angert lab group at UBC we have been working with the riparian herb Mimulus cardinalis (the scarlet monkeyflower) as a case study system to compare predictions from correlative niche models with experimental field studies. Earlier studies that transplanted the species along its elevational range limit found a sharp fitness decline beyond its upper elevational range limit consistent with niche model predictions, but wild populations growing near the upper elevational range limit did not necessarily have lower fitness. From demographic surveys across the entire latitudinal extent of this species, it has been difficult to associate population performance with climatic suitability inferred from the niche models. Last summer we carried out a field transplant study along the species northern range limit and preliminary results seem to provide evidence for dispersal limitation. By integrating results from field experiments and population ecology we can understand scenarios that may lead to faulty predictions from correlative niche model. We can then develop approaches for other species to make more accurate forecasts of range shifts with climate change.
Figure 1: Geographic distribution of Mimulus cardinalis (the scarlet monkeyflower) across Oregon and California. Dots are record localities and colour-shaded portions highlight regions of high predicted suitability from climatic niche models.
Figure 2: Typical stream habitat and field sites for Mimulus cardinalis (the scarlet monkeyflower).
Professor Emeritus
Instructor Emeritus
Instructor Emeritus
Professor Emeritus
Professor Emeritus
Professor Emeritus
Professor Emeritus
Academic History
Contact Information
Physiology, biochemistry, and molecular genetics of inorganic ion transport across membranes of plant roots. Physiological aspects of hydroponic growth.
Research Interests
Plant nutrition involves the acquisition of the essential chemical elements, and their utilization for growth and development. Research in my laboratory is focussed upon membrane transport processes responsible for transferring inorganic ions (particularly K+, NH4+ and NO3-) across the plasma membrane, from external (soil) solution, into the roots of higher plants.
We are studying these transport processes, with emphasis on NO3- and NH4+ transport, at the molecular level (cloning genes and examining the results of gene deletions), through the physiological to ecological levels. In the case of NO3-, substantial quantities of this ion are transported to the leaves of many plants where it is reduced to NH4+ and converted to amino acids and other N compounds. Thus, ion uptake is not exclusively a function of root cells only, but leaves too must reabsorb nitrate from the xylem sap.
The basic approach that my group is undertaking is to integrate physiological, biochemical and molecular studies of the transport of NO3- and NH4+ across the plasma membranes of root cells. Genes encoding high- and low-affinity NO3- transporters and high-affinity NH4+ transporters have been cloned by my group, and we are attempting to resolve the manner in which the proteins encoded by these genes participate in transport processes, and the manner in which they are regulated.
In Arabidopsis thaliana there are a total of eleven genes encoding NO3- transporters and 5 encoding NH4+ transporters. We are attempting by use of T-DNA insertional mutants and other methods to determine the function of each of these transporters. Given the complexity of this large number of genes we are also working with a relatively much simpler fungal system, Aspergillus nidulans, where there are only two NO3- transporters. We have cloned both of the genes encoding these transporters and are trying to work out the functional significance of the two transporters.
Selected Publications
Britto, DT, MY Siddiqi, ADM Glass, & HJ Kronzucker. 2001. Futile membrane ion cycling: a new cellular hypothesis to explain ammonium toxicity in plants. PNAS 98: 4255-4258.
Glass, ADM, DT Britto, J Kaiser, HJ Kronzucker, A Kumar, M Okamoto, MY Siddiqi & JJ Vidmar. 2001. The regulation of nitrate and ammonium transport systems in plants. J. Exp. Bot. In press.
Unkles, SE, D Zhou, MY Siddiqi, JR Kinghorn & ADM Glass. 2001. Apparent genetic redundancy facilitates ecological plasticity for nitrate transport. EMBO J. 20: 6246-6255.
Vidmar, J, D Zhuo, MY Siddiqi, JK Schjoerring, B Touraine, & ADM Glass. 2000. Regulation of HvNRT2 expression and high-affinity nitrate influx in roots of Hordeum vulgare by ammonium and amino acids. Plant Physiology 123: 307-318.
Professor Emeritus
Academic History
Contact Information
Genomics, molecular evolution and chloroplast protein import in algae with chlorophyll c (diatoms, dinoflagellates and other chromists). Evolution of genes for light-harvesting antenna proteins and their stress-induced relatives. Photoacclimation in diatoms. Replication and transcription of minicircular chloroplast genes in dinoflagellates.
Research Interests
All my research is concerned with chloroplasts, the photosynthetic organelles of eukaryotic cells. It brings together the structure of photosynthetic membrane proteins with the evolution of the gene families that encode them, as well as the evolutionary origins of the chloroplasts themselves. We are currently investigating the amazing minicircular genes found in dinoflagellate chloroplasts‹each gene on its own individual minicircle of DNA, “one gene-one circle”! These were the first chloroplast gene sequences ever reported for dinoflagellates, a large group of unicellular marine algae.
Another project concerns how nuclear-encoded proteins are imported into the chloroplasts of chromophyte (Chl a/c-containing) algae, which are bounded by four envelope membranes rather than two. Both these groups of algae are believed to have originated by secondary endosymbiosis, where a non-photosynthetic eukaryote engulfed a photosynthetic eukaryote rather than a prokaryote. We are looking for molecular clues about how this process might have occurred.
Most of my earlier research concerned the extended family of chlorophyll (Chl) a/b and Chl a/c light-harvesting proteins in plants and algae. Now we are looking more closely at the Chl a/c family of the chromophyte alga Heterosigma, to try to determine if there is preferential association of any particular members of this family with Photosystem I or Photosystem II. From phylogenetic trees, it is clear that the Chl a/b and the Chl a/c proteins diverged from each other early in the evolution of the photosynthetic eukaryotic cell, so the organization of the chlorophyll a/c protein complexes in the thylakoid membrane may be quite different.
Selected Publications
Books and Chapters:
B.R. Green (2007) Evolution of light-harvesting antennas in an oxygen world. In:“Evolution of Aquatic Photoautotrophs”. P.G. Falkowski and A.H. Knoll (Editors). Academic Press, in press.
B.K Chaal and B.R. Green (2007) Protein targeting in “Secondary” or “Complex” Chloroplasts. In: “Protein Targeting Protocols: 2nd Edition”. M. van der Giezen (Editor). Humana Press, in press.
B. R. Green and E. Gantt (2005) Distal Antennas of Photosystem II. In “Photosystem II: The Water/Plastoquinone Oxido-Reductase In Photosynthesis”. T. Wydrzynski and K. Satoh (Editors). Kluwer Academic Press, Dordrecht.
“Light-harvesting Antennas in Photosynthesis” (2003) Eds. B. R. Green and W. W. Parson. Kluwer Academic Press, Dordrecht. Authored two chapters of that book:
B. R. Green, J.A. Anderson, W.W. Parson (2003) Photosynthetic membranes and their light-harvesting antennas. Ibid, pp 1-28
B. R. Green (2003) The evolution of light-harvesting antennas. Ibid, pp129-168
Papers
Martha J. Nelson, Yunkun Dang, Elena Filek, Zhaoduo Zhang, Vionnie Yu, Ken-ichiro Ishida and Beverley R. Green. (2007) Identification and transcription of transfer RNA genes in dinoflagellate plastid minicircles. Gene 392: 291-298.
Marie-Pierre Oudot-Le Secq, Jane Grimwood, Harris Shapiro, E.Virginia Armbrust, Chris Bowler and Beverley R. Green. (2007) Chloroplast genomes of the diatoms Phaeodactylum tricornutum and Thalassiosira pseudonana: Comparison with other plastid genomes of the red lineage. Mol. Gen. Gen. 277:427–439.
M. Oborník and B.R. Green. (2005) “Mosaic Origin of the Heme Biosynthesis Pathway in Photosynthetic Eukaryotes”. Mol. Biol. Evol. 22: 2343-2353.
M. J. Nelson and B.R.Green. (2005) “Double hairpin elements and tandem repeats in the non-coding region of Adenoides eludens chloroplast gene minicircles.” Gene 358: 102-110.
P. Juneau, B. R. Green and P. J. Harrison. (2005) Simulation of pulse-amplitude-modulated (PAM) fluorescence: Limitations of some PAM-parameters in studying environmental stresss effects. Photosynthetica 43: 75-83.
B.K. Chaal and B.R. Green. (2005) “Protein import pathways in ‘complex’ chloroplasts derived from secondary endosymbiosis involving a red algal ancestor.” Plant Molecular Biology 57: 333-342.
F. Bentley, J. Harnett, K. Ishida and B.R.Green (2005) “Investigation of PSI-associated light-harvesting proteins in a chromophyte alga.” In: Photosynthesis: Fundamental Aspects to Global Perspectives (Eds. A. van der Est and D. Bruce), pp.161-163.
E.V.Armbrust, J.A.Berges, C. Bowler, B.R. Green, D. Martinez and 38 others. (2004) “The genome of the diatom Thalassiosira pseudonana: ecology, evolution, and metabolism”. Science 306: 79-86
B.R. Green (2004) The chloroplast genome of dinoflagellates – A reduced instruction set? Protist 155: 23-31
E. L. Camm and B.R. Green (2004) How the chlorophyll-proteins got their names. Photosyn Res 80: 189-196
B. K. Chaal, K. Ishida and B.R.Green (2003) A thylakoidal processing peptidase from the heterokont alga Heterosigma akashiwo. Plant Mol Biol 52: 463-472
K. Ishida and B.R. Green (2002) Second- and third-hand chloroplasts in dinoflagellates: Phylogeney of oxygen-evolving enhancer 1 (PsbO) protein reveals replacement of a nuclear-encoded plastid gene by that of a haptophyte tertiary endosymbiont. Proc Natl Acad Sci USA 99: 9294–9299
Z. Zhang, T. Cavalier-Smith and B.R.Green (2002)“Evolution of dinoflagellate unigenic minicircles and the partially concerted divergence of their putative replicon origins.” Mol Biol Evol 19: 489-500
Z. Zhang, T. Cavalier-Smith and B.R. Green (2001) “A family of selfish minicircular chromosomes with jumbled chloroplast gene fragments from a dinoflagellate.” Mol Biol Evol 18:1558-65
B.R. Green (2001) “Was ‘molecular opportunism’ a factor in the evolution of different photosynthetic light-harvesting pigment systems?” Proc. Natl. Acad. Sci. USA 98: 2119-2121
Z. Zhang, B.R. Green and T. Cavalier-Smith (2000) “Phylogeny of Ultra-rapidly Evolving Dinoflagellate Chloroplast Genes: A Possible Common Origin for Spoorozoan and Dinflagellate Plastids.” J. Mol. Evol. 51: 26-40
K. Ishida, T. Cavalier-Smith and B.R.Green (2000) “Endomembrane Structure and the Chloroplast protein Targeting Pathway in Heterosigma akashiwo (Raphidophyceae, Chromista). J. Phycol. 36; 1135-1144
B.R. Green and E. Gantt (2000) “Is Photosynthesis Really Derived from Purple Bacteria?”. J. Phycol.36: 983-985
Z. Zhang, B.R.Green and T. Cavalier-Smith (1999) “Single Gene Circles in Dinoflagellate Chloroplast Genomes” Nature 400: 155-159
K. Ishida, B.R.Green and T. Cavalier-Smith (1999) “Diversification of a Chimaeric Algal Group, the Chlorarachniophytes: Phylogeny of Nuclear and Nucleomorph Small Subunit RRNA Genes.” Mol. Biol. Evol. 16: 321-331.
D.G. Durnford, J.A. Deane, S. Tan, G.I. McFadden, E. Gantt, B.R.Green, (1999) “A Phylogenetic Assessment of Eukaryotic Light-harvesting Antenna Proteins, with implications for Plastid Evolution”, J. Mol. Evol. 48: 59-68.
B.R. Green, S. Singh, I Babic, C. Bladen, A. Johnson-Flanagan, (1998)“Relationship of chlorophyll, seed moisture and ABA levels in the maturing Brassica seed and effect of a mild freezing stress”. Physiol. Plant. 104: 125-133.
B.R. Green (1998) “Solutions to the Light-harvesting Problem: Mix, Match and Duplicate”. (Invited Symposium talk), published in Proceedings of XIth International Congress on Photosynthesis, Budapest, Aug. 1998.
G.W.M. van der Staay, N. Yurkova, B.R.Green (1998) “The 38 kDa Chlorophyll a/b Protein of the Prokaryote Prochlorothrix hollandica is Encoded by a Divergent pcb Gene”, Plant Mol. Biol. 36: 775-783.
R.D.Dinkins, H. Bandaranayake, L. Baeza, A.J.F. Griffiths, B.R. Green (1997) “hcf5, a Nuclear Photosynthetic Electron Transport Mutant of Arabidopsis thaliana with a Pleiotropic Effect on Chloroplast Gene Expression”. Plant Physiol. 113: 1023-1031 (and on the cover!).
B.R. Green and D. Durnford (1996) “Chlorophyll-carotenoid Proteins in Oxygenic Photosynthesis”. Annu. Rev. Plant Physiology, Plant Mol. Biol. 47:685-714.
J. La Roche, G.W.M. van der Staay, F. Partensky, A. Ducret, R. Aebersold, R. Li, S.S.Golden, R.G.Hiller, P.M. Wrench, A.W.D.Larkum, B.R.Green (1996) “Independent Evolution of the Prochlorophyte and Green Plant Chlorophyll a/b Light-harvesting Proteins” Proc. Natl. Acad. Sci. USA 93: 15244-15248.
D.G. Durnford, R. Aebersold, B.R.Green (1996) “The Fucoxanthin-chlorophyll Proteins from a Chromophyte Alga Are Part of a Large Multigene Family: Structural and Evolutionary Relationships to Other Light Harvesting Antennae” Mol. Gen. Genet. 253: 377-386.
Professor Emeritus
Professor Emeritus
Professor Emeritus
Academic History
Contact Information
My Links
Haughn laboratory web page: http://haughnlab.botany.ubc.ca
Research Interests
My laboratory uses molecular genetics and the seed coat epidermal cell of Arabidopsis thaliana as a model to investigate plant cell wall biosynthesis, structure and function. We also run a TILLING service described below.
Mechanisms underlying cell differentiation. The seed coat is a specialized tissue derived from ovule integuments. In some species, including Arabidopsis thaliana, large quantities of polysaccharide mucilage (pectin) and secondary cell wall are produced by seed coat epidermal cells at specific times during differentiation. Because these modifications are not required for viability, the Arabidopsis seed coat epidermal cells represent a unique dispensable tissue that can be used to identify genes involved in complex polysaccharide biosynthesis and secretion (Haughn and Chaudhury, 2005; Arsovski et al., 2010; Haughn and Western, 2012; Sôla et al., 2019a), important processes that are incompletely understood. As a first step to develop this unique model system we investigated the structure and differentiation of Arabidopsis seed coat secretory cells including the synthesis, secretion and extrusion of mucilage (Western et al., 2000) and isolated a number of mutants defective in the differentiation, synthesis and extrusion of mucilage (Western et al., 2001). Our characterization of these and other mutants and cloning of the mutated genes has identified a number of proteins playing key roles in cell wall biology including biosynthesis (MUM4; Western et al., 2004) modification (MUM2, BXL1, FLY1, SOS5, HMS, FLY2, RUBY; Dean et al., 2007, Arsovski et al., 2009, Voiniciuc et al., 2013, Griffiths et al., 2014, Levesque-Tremblay et al., 2015, Kunieda et al., 2019; Sôla et al., 2019a) secretion (Young et al., 2008; McFarlane et al., 2013; Shi et al., 2019) and regulation (Western et al., 2004; Huang et al., 2011; Bargava et al., 2013) of mucilage pectins and synthesis of cellulose in mucilage (CESA5; Mendu et al., 2011; Griffiths et al., 2015) and for the secondary cell wall columella (CESA2, CESA5, CESA9; Stork et al., 2009, Mendu et al., 2011). We have also developed some useful information and tools for the study of the seed coat including a microarray analysis (Dean et al., 2011); mucilage proteomic analysis (Tsai et al., 2017) and seed coat specific promoters (Esfandiari., 2013; Dean et al., 2017; Tsai et al., 2017; McGee et al., 2019) and used the information to engineer mucilage in situ (McGee et al., 2021). Finally, we have discovered a metabolic link between the production of mucilage and the synthesis of storage oil in the embryo (Shi et al., 2012). Due to my upcoming retirement I am no longer initiating new projects.
Selected Publications
McGee, R., G.H. Dean, D. Wu, Y. Zhang, S.D. Mansfield, G.W. Haughn. 2021. Pectin Modification in Seed Coat Mucilage by In Vivo Expression of Rhamnogalacturonan-I- and Homogalacturonan-Degrading Enzymes Plant Cell Physiol., in press, https://doi.org/10.1093/pcp/pcab077
Šola, K., G.H. Dean and G.W. Haughn. 2019. Arabidopsis seed mucilage: a specialised extracellular matrix that demonstrates the structure–function versatility of cell wall polysaccharides Ann. Plant Rev. 2: 1085-1116, doi: 10.1002/9781119312994.apr0691
Kunieda, T., I. Hara-Nishimura, T. Demura and G.W. Haughn. 2019. Arabidopsis FLYING SAUCER2 functions redundantly with FLY1 to establish normal seed coat mucilage. Plant Cell Physiol. 61: 308-317, https://doi.org/10.1093/pcp/pcz195
Shi, L., G. Dean, H. Zheng, M.J. Meents, T.M. Haslam, G.W. Haughn, L. Kunst. 2019. ECERIFERUM11/C-TERMINAL DOMAIN PHOSPHATASE LIKE 2 affects secretory trafficking. Plant Physiol. 181: 901–915. doi: 10.1104/pp.19.00722
McGee, R., G. Dean, S.D. Mansfield and G.W. Haughn. 2019. Assessing the utility of seed coat-specific promoters to engineer cell wall polysaccharide composition of mucilage. Plant Mol. Biol. 101:373-387 DOI: 10.1007/s11103-019-00909-8
Šola, K, E.J. Gilchrist, D. Ropartz, L.Wang, I. Feussner, S.D. Mansfield, M.-C. Ralet, and G.W. Haughn. 2019. RUBY, a putative galactose oxidase, influences pectin properties and promotes cell-to-cell adhesion in the seed coat epidermis of Arabidopsis thaliana. Plant Cell: 31: 809-831 (Faculty 1000 Recommended).
Dean, G.H., Z. Jin, L. Shi, E. Esfandiari, R. McGee, K. Nabata, T. Lee, L. Kunst1, T. L. Western and G.W. Haughn. 2017.Identification of a seed coat-specific promoter fragment from the Arabidopsis MUCILAGE-MODIFIED4 gene. Plant Mol. Biol. 95: 33-50.
Tsai, A.Y.-L., T. Kunieda, J. Rogalski, L.J. Foster, B.E. Ellis and G.W. Haughn. 2017. Identification and Characterization of Arabidopsis Seed Coat Mucilage Proteins. Plant Physiol. 173: 1059–1074.
Griffiths J.S., K. Šola, R. Kushwaha, P. Lam, M. Tateno, R. Young, C. Voiniciuc, G. Dean, S.D. Mansfield, S. DeBolt, and G.W. Haughn. 2015. Unidirectional movement of cellulose synthase complexes in Arabidopsis seed coat epidermal cells deposit cellulose involved in mucilage extrusion, adherence and ray formation. Plant Physiol. 168: 502-520.
Levesque-Tremblay, G., K. Müller, S.D. Mansfield, G.W. Haughn. 2015. HIGHLY METHYL ESTERIFIED SEEDS is a Pectin Methyl Esterase involved in embryo development. Plant Physiol. 167: 725-737.
Griffiths, J.S., A. Y.-L. Tsai, H. Xue, , C. Voiniciuc, K. Šola, G.J. Seifert, S.D. Mansfield and G.W. Haughn. 2014. SOS5 mediates Arabidopsis seed coat mucilage adherence and organization through pectins. Plant Physiol. 165: 991-1004.
Voiniciuc C., G.H. Dean, J.S. Griffiths, K. Kirchsteiger, Y-T. Hwang, A. Gillett, G. Dow, T.L. Western, M. Estelle, and G.W. Haughn. 2013. FLYING SAUCER1 Is a Transmembrane RING E3 Ubiquitin Ligase That Regulates the Degree of Pectin Methylesterification in Arabidopsis Seed Mucilage. Plant Cell 25: 944-959 (cover article).
Esfandiari E., Z. Jin, A. Abdeen, J.S. Griffiths, T.L. Western and G.W. Haughn. 2013. Identification and analysis of an outer-seed-coat-specific promoter from Arabidopsis thaliana. Plant Mol. Biol. 81:93–104.
Haughn G.W. and T.L. Western. 2012. Arabidopsis seed coat mucilage is a specialized cell wall that can be used as a model for genetic analysis of plant cell wall structure and function. Frontiers Plant Sci. 3: 64.
Dean G., Y. Cao, D Xiang, N.J. Provart, L. Ramsay, A. Ahad, R. White, G. Selvaraj, R. Datla and G. Haughn. 2011. Analysis of gene expression patterns during seed coat development in Arabidopsis. Mol. Plant 4: 1074-1091.
Huang J., D. DeBowle2, E. Esfandiari1, G. Dean, N.C. Carpita and G.W. Haughn. 2011. The Arabidopsis Transcription Factor LUH/MUM1 Is Required for Extrusion of Seed Coat Mucilage. Plant Physiol: 156: 491-502.
Arsovski, A.A., G.W. Haughn and T.L. Western. 2010. Seed coat mucilage cells of Arabidopsis thaliana as a model for plant cell wall research. Plant Signaling & Behavior 5: 796 – 801.
Arsovski A.A., T.M. Popma, G.W. Haughn, N.C. Carpita, M.C. McCann and T.L. Western. 2009. AtBXL1 encodes a bifunctional β-D-xylosidase/α-L-arabinofuranosidase required for pectic arabinan modification in Arabidopsis thaliana mucilage secretory cells. Plant Physiol. 150: 1219-1234.
Young, RE, HE McFarlane, MG Hahn, TL Western, GW Haughn, and AL Samuels. (2008). Analysis of the Golgi Apparatus in Arabidopsis Seed Coat Cells during Polarized Secretion of Pectin-Rich Mucilage. Plant Cell 20: 1623–1638.
Dean G.H., H. Zheng, J. Tewari, J. Huang, D. S. Young, Y.T. Hwang, T. L. Western, N.C. Carpita, M.C. McCann, S.D. Mansfield, and G. W. Haughn. (2007). The Arabidopsis MUM2 Gene Encodes a ß-Galactosidase Required for the Production of Seed Coat Mucilage with Correct Hydration Properties. Plant Cell 19: 4007-4021.
Haughn G. and A. Chaudhury. (2005). All Dressed Up With Nowhere To Go: Genetic Analysis of Seed Coat Development in Arabidopsis. Trends in Plant Science 10: 472-477.
Western T.L., 2, D.S. Young, G.H. Dean, W.L. Tan, A.L. Samuels, and G.W. Haughn (2004) MUCILAGE-MODIFIED4 Encodes a Putative Pectin Biosynthetic Enzyme Developmentally Regulated by APETALA2, TRANSPARENT TESTA GLABRA1, and GLABRA2 in the Arabidopsis Seed Coat. Plant Physiology, 134: 296–306
Western, T.L., J. Burn, W. L. Tan, D. Skinner, L. M. McCaffrey, B. Moffatt and G. W. Haughn. (2001) Isolation and characterization of mutants defective in seed coat mucilage secretory cell development in Arabidopsis . Plant Physiology 127: 998-1011.
Western, TL, DJ Skinner & GW Haughn. (2000). Differentiation of mucilage secretory cells of the Arabidopsis thaliana seed coat. Plant Physiology 122: 345-355.
Senior Instructor Emeritus
Academic History
Contact Information
Teaching & Learning, Systematics & Phylogenetics, Marine Biology, Algae.
Research Interests
I have broad natural history interests with a special fondness for marine, mountain, and desert ecosystems. Since 1972 my marine research has focused on the seaweed flora of British Columbia (natural history, systematics, and reproductive biology). Part of this work has involved conducting intertidal and subtidal surveys along many portions of the British Columbia coast as well as in Washington, California, New Zealand and Australia. I spent three years working for the University of Auckland’s Leigh Marine Laboratory.
As a biologist I am especially concerned with loss of organismal diversity, and with ecosystem services. I have therefore taken an active role in conservation issues, especially the status of marine protected areas in British Columbia.
I have a general interest in natural history and broad background in organismal diversity; as a result my departmental teaching has focused on introductory and organismal courses. I am especially concerned that students gain an appreciation for organisms in the context of their environments and to this end attempt to give them field experience wherever possible.
My marine research interests are in the following areas: 1. The natural history, biogeography, and systematics of Pacific Northwest & New Zealand seaweed floras; 2. Reproductive biology of red algae, and 3. The role of marine protected areas (MPA’s) in coastal zone conservation strategies.
At the drier-plant end of the spectrum, I have a keen interest in the biology and horticulture of succulent plants (especially those on C.I.T.E.S. Appendix I & II).
Selected Publications
Hawkes, M.W. 2005. In search of cacti and seaweeds on desert shores: E. Yale Dawson (1918-1966), Botanist. Haseltonia (Scientific Yearbook of the Cactus & Succulent Society of America) 11: 126-137. [PDF]
Hawkes, M.W. collaborated on the seaweed section of: Lamb, A. & B. Hanby 2005. Marine Life of the Pacific Northwest. Harbour Publishing: Madeira Park, B.C. [Title Page]
Shepherd, S.A. & M.W. Hawkes. 2005. Algal food preferences and seasonal foraging strategy of the marine iguana, Amblyrhynchus cristatus, on Santa Cruz, Galápagos. Bulletin of Marine Science 77: 51-72. [PDF]
Hawkes, M.W. 2003. Cactaceae contributions for: Pereskioideae, Pereskia, Hylocereus, Selenicereus, and Epiphyllum, In: Flora of North America north of Mexico. Vol. 4. Magnoliophyta: Caryophyllidae, part 1. Oxford Univ. Press: New York, Oxford.
Gabrielson, P.G., T.B. Widdowson, S.C. Lindstrom, M.W. Hawkes, & R.F. Scagel. 2000. Keys to the benthic marine algae and seagrasses of British Columbia, southeast Alaska, Washington and Oregon. Phycological Contribution No. 5, Dept. of Botany, Univ. of B.C.: Vancouver. [Order]
Nelson, W.A., G.A. Knight, & M.W. Hawkes. 1998. Porphyra lilliputiana sp. nov. (Bangiales, Rhodophyta) – a diminutive New Zealand endemic with novel reproductive biology. Phycological Research 46: 57-61.
Hawkes, M.W. 1994. Conserving Marine Ecosystems: Are British Columbia’s Marine Protected Areas Adequate? Chap. 28 In: Biodiversity in British Columbia: Our Changing Environment, edited by E. McCullum & L. Harding. Environment Canada: Vancouver.
Scagel, R.F., P.W. Gabrielson, D.J. Garbary, L. Golden, M.W. Hawkes, S.C. Lindstrom, J.C. Oliveira & T.B. Widdowson. 1993. A Synopsis of the Benthic Marine Algae of British Columbia, Southeast Alaska, Washington and Oregon. Phycological Contribution no. 3: vi + 535 pp. Dept. of Botany, University of British Columbia: Vancouver.
Professor Emeritus
Academic History
Contact Information
My Links
Research Interests
Fatty acids and lipids are essential components of plant cells with diverse structural and signaling functions. They also form waxy cuticles on the plant surface required for plant protection against water loss, pathogens and insects, and serve as storage reserves in the seed that are exploited for human nutrition, used as industrial feedstocks, lubricants, and fuel. Research in my lab is directed toward understanding the following aspects of plant fatty acid and lipid metabolism:
1. Biosynthesis and secretion of cuticular wax. The cuticle is a thin hydrophobic layer which covers the outermost surface of the primary aerial tissues of land plants. It protects plants from uncontrolled water loss, UV radiation, as well as bacterial and fungal pathogens. It also mediates a variety of plant interactions with insects and prevents fusions between plant organs during development. The cuticle is synthesized by epidermal cells and its framework is provided by cutin, insoluble plant-specific polyester composed of C16 and C18 hydroxy and epoxy fatty acids and glycerol. The cutin matrix is embedded in and overlaid with cuticular waxes, complex mixtures of mostly very-long-chain fatty acid (VLCFA) derivatives easily extractable by organic solvents.
Similar wax constituents comprising cuticular wax of most plant species suggest that the basic mechanisms involved in wax production and transport to the cuticle are highly conserved in the plant kingdom, but our understanding of these processes is limited. We are using wax-deficient eceriferum mutants, standard reverse genetic techniques, as well as genomic chemical and molecular cell approaches in Arabidopsis thaliana to:
• identify and functionally characterize gene products involved in wax biosynthesis and secretion
• gain insights into regulation of wax deposition in epidermal cells
• establish subcellular localization of the enzymes of wax biosynthesis, and
• determine the mechanism of wax transport to the cuticle
2. Regulation of seed storage oil production. As the pathways of oil biosynthesis in seeds are becoming better understood it is clear that total seed oil content can be altered by the manipulation of transcription factors that control plant oil biosynthesis, or manipulation of specific enzymes involved in this process. Oil biosynthetic enzymes can be broadly divided into two categories: those controlling fatty acid biosynthesis (source), and those involved in storage triacylglycerol (TAG) assembly (sink). This project is aimed at identifying key regulators and enzymes determining the seed oil content in Arabidopsis thaliana and their relative contributions to the overall levels of oil accumulation.
Selected Publications
P. Lam, L. Zhao, N. Eveleigh, Y. Yu, X. Chen, and L. Kunst (2014) The exosome and trans-acting siRNAs regulate cuticular wax biosynthesis during Arabidopsis inflorescence stem development. Plant Physiol. Dec. 11: [Epub ahead of print].
C. Nawrath, L. Schreiber, R. Franke, N. Geldner, J. J. Reina-Pinto and L. Kunst (2013) Apoplastic Diffusion Barriers in Arabidopsis thaliana. The Arabidopsis Book 2013 (http://www.bioone.org/doi/pdf/10.1199/tab.0167)
T. Roscoe and L. Kunst (2013) Seed Power: Increasing Oil Content by Redirecting Carbon Flux During Development. International Innovation Article 2013
T. M. Haslam and L. Kunst (2013) Extending the story of very-long-chain fatty acid elongation. Plant Science 210: 93– 107.
T. M. Haslam and L. Kunst (2013) Wax Analysis of Stem and Rosette Leaves in Arabidopsis thaliana. Bio-Protocols. (http://www.bio-protocol.org/wenzhang.aspx?id=782)
E. Sakuradani, L. Zhao, T. M. Haslam and L. Kunst (2012) The CER22 gene required for the synthesis of cuticular wax alkanes in Arabidopsis thaliana is allelic to CER1. Planta 237:731–738.
T.M. Haslam, A. Mañas Fernández, L. Zhao, and L. Kunst (2012) Arabidopsis ECERIFERUM2 is a component of the fatty acid elongation machinery required for fatty acid extension to exceptional lengths. Plant Physiol. 160: 1164-1174.
P. Lam, L. Zhao, H.E. McFarlane, M. Aiga, V. Lam, T.S. Hooker, and L. Kunst (2012) RDR1 and SGS3, components of RNA-mediated gene silencing, are required for regulation of cuticular wax biosynthesis in developing stems of Arabidopsis. Plant Physiol. 159: 1385-1395.
Shi, L., Katavic, V., Yu, Y., Kunst, L. and Haughn, G. (2012) Arabidopsis glabra2 mutant seeds deficient in mucilage biosynthesis produce more oil. Plant J. 69, 37-46.
L. Zhao, V. Katavic, F. Li, G. W. Haughn, and L. Kunst (2010) Insertional mutant analysis reveals that LONG-CHAIN ACYL-COA SYNTHETASE 1 (LACS1), but not LACS8, functionally overlaps with LACS9 in Arabidopsis seed oil biosynthesis. Plant J. 64, 1048-1058.
L. Kunst and L. Samuels (2009) Plant cuticles shine: advances in wax biosynthesis and export. Curr. Opin. Plant Biol. 12: 721-727
X. Wu, F. Beaudoin, F. Li, R. P. Haslam, J. E. Markham, H. Zheng, J. A. Napier and L. Kunst (2009) Functional characterization of the Arabidopsis thaliana β-ketoacyl-CoA reductase candidates of the fatty acid elongase. Plant Physiology 150:1174-1191.
A. DeBono, T. Yeats, J.K.C. Rose, D. Bird, R. Jetter, L. Kunst and A.L.Samuels (2009) LTPG is a glycosylphosphatidylinositol-anchored lipid transfer protein required for export of lipids to the plant surface. Plant Cell 21: 1230-1238.
F. Li, X. Wu, P. Lam, D. Bird, H. Zheng, L. Samuels, R. Jetter and L. Kunst (2008) Identification of the wax ester synthase/acyl-coenzyme A:diacylglycerol acyltransferase WSD1 required for stem wax ester biosynthesis in Arabidopsis. Plant Physiol. 148:97-107.
R. Jetter and L. Kunst (2008) Plant surface lipid biosynthetic pathways and their utility for metabolic engineering of waxes and hydrocarbon biofuels. Plant J. 54: 670–683.
L. Samuels, L. Kunst, and R. Jetter (2008) Sealing plant surfaces: Cuticular wax formation by epidermal cells. Annu. Rev. Plant Biol. 59: 683-707.
S. Greer, M. Wen, D. Bird, X. Wu, L. Samuels, L. Kunst, and R. Jetter (2007) The cytochrome P450 enzyme CYP96A15 is the mid-chain alkane hydroxylase responsible for formation of secondary alcohols and ketones in stem cuticular wax of Arabidopsis thaliana. Plant Physiol. 145: 653-667.
O. Rowland, R. Lee, R. Franke, L. Schreiber and L. Kunst (2007) The CER3 gene from Arabidopsis thaliana is allelic to WAX2/YRE/FLP1 and is required for cuticular wax biosynthesis. FEBS Lett. 581: 3538–3544.
D. Bird, F. Beisson, A. Brigham, J. Shin, S. Greer, R. Jetter, L. Kunst, X. Wu, A. Yephremov, and L. Samuels (2007) Characterization of Arabidopsis ABCG11/WBC11, an ATP binding cassette (ABC) transporter that is required for cuticular lipid secretion. Plant J. 52: 485-498.
C. Lai, L. Kunst and R. Jetter (2007) Composition of alkyl esters in the cuticular wax on inflorescence stems ofArabidopsis thaliana cer mutants. Plant J. 50: 189-196.
T. S. Hooker, P. Lam, H. Zheng, and L. Kunst (2007) A core subunit of the RNA-processing/degrading exosome specifically influences cuticular wax biosynthesis in Arabidopsis. Plant Cell 19: 904–913.
O. Rowland, H. Zheng, S.R. Hepworth, P. Lam, R. Jetter, and L. Kunst (2006) CER4 encodes an alcohol-forming fatty acyl-coenzyme A reductase involved in cuticular wax production in Arabidopsis. Plant Physiol. 142: 866-877.
M.C. Suh, A. L. Samuels, R. Jetter, L. Kunst, M. Pollard, J. Ohlrogge, and F. Beisson (2005) Cuticular lipid composition, surface structure, and gene expression in Arabidopsis stem epidermis. Plant Physiol. 139: 1649–1665.
H. Zheng, O. Rowland, and L. Kunst (2005) Disruptions of the Arabidopsis enoyl-CoA reductase gene reveal an essential role for very-long-chain fatty acid synthesis in cell expansion during plant morphogenesis. Plant Cell 17: 1467-1481.
H. Moon, G. Chowrira, O. Rowland, B. J.Blacklock,M. A. Smith, and L. Kunst (2004) A root-specific condensing enzyme from Lesquerella fendleri that elongates very-long-chain saturated fatty acids. Plant Mol. Biol. 56: 917-927.
J. A. Pighin, H. Zheng, L. J. Balakshin, I. P. Goodman, T. L. Western, R. Jetter, L. Kunst, and A. L. Samuels (2004) Plant cuticular lipid export requires an ABC transporter. Science 306: 702-704.
Figure 1. Five years old Populus trichocarpa trees at Totem Field collection, UBC.
Article by Adriana Suarez-Gonzalez (Douglas lab)
If you are reading this article, then you survived your birth, acclimated to rainy and cold weather and have successfully battled a number of flu viruses. The same happens in trees, but because plants are stationary and can’t move, these issues are even more extreme. When a seed lands on the soil it has two options: survive or die – it can’t move to a more favorable environment. Just as hybrid cars use two or more distinct power sources to move the vehicle (usually a combination of an internal combustion engine and an electric car), so some tree species can form hybrids when two different species cross. Also like hybrid cars, hybrid trees are beneficial and could help mitigate the effects of climate change. Hybrid trees grow faster than their parents and are more resistant to extreme environments (this is called ‘hybrid vigor’). Thus the hybrids have better survival which makes them attractive to the forestry industry where they can be used for carbon sequestration and biofuel production. However, to make this a reality and to select the best hybrid varieties we need to understand why hybrid trees perform better than their parents. For hybrid cars, we understand their engineering and why they are environmental friendly and efficient. But when it comes to the ‘engineering’ behind hybrid trees, it is not so clear. By investigating their genomes and the letters in their DNA we will find interesting clues to better understand hybrid vigor.
I studied the genome of 200 trees from two species of trees that form hybrids: black cottonwood (Populus trichocarpa) and balsam poplar (P. balsamifera). In 3 chromosomes, from beginning to end, I searched each letter of DNA and found a special segment of the genome that caught my attention. I found this segment in black cottonwood but it originated from balsam poplar. In addition, this segment of the genome was loaded with genes and information crucial for survival and adaptation. It was interesting to discover how choosy black cottonwoods are; while mating with balsam poplars they keep the ‘good’ balsam genes. Are black cottonwoods actually selecting this region of the balsam genome? Black cottonwood grows from California, where is warm, to Alaska, while balsam poplar is found at higher latitudes from Alaska to Newfoundland, where winters are long and harsh. So the question arises if black cottonwoods with balsam genes are more resistant to cold compared to black cottonwoods without these balsam genes? This is the question I will be addressing in the upcoming months. I also plan to explore the 85% of the genome from these two poplars patiently waiting for me in my computer; so far I have analyzed 3 of the 19 chromosomes present. The results of this research will advance our knowledge in understanding why hybrid trees perform better than their parents and help us optimize tree plantations in the face of climate change.
Figure 2. Phenotypic variation in Populus trichocarpa from northern and southern locations. Trees from the north (left) lose their leaves earlier in the fall compared to trees from southern populations (right). This could be an adaptation to earlier winters at higher latitudes.
Figure 3. Rows of Populus trichocarpa trees at Totem Field collection, UBC.
Figure 4. During May and June I visited the Populus trichocarpa Totem Field collection at UBC field and sampled young leaves, froze them in liquid nitrogen and brought them back to the lab to extract DNA.
Article by Bill Harrower, Jenny McCune, and Jeannette Whitton
National level endangered species laws are designed to prevent species from going extinct. Canada’s endangered species law, the Species At Risk Act (or SARA), was enacted almost 13 years ago1. Associate Professor Jeannette Whitton led a group of 14 students and researchers from the UBC Botany Department, the UBC Department of Zoology, Simon Fraser University and the Beaty Biodiversity Museum has worked to understand what threatens species at risk in Canada, and how recovery planning is proceeding for these species.
Once a species is listed, SARA outlines a specific process with mandated timelines. Recovery strategies are to be finalized within one to two years of a species being listed under the law, depending on whether a species is listed as threatened or endangered. Recovery strategies must be publically available and must include three things. They must identify the threats to a species’ survival, state specific objectives for recovery, and define the area that contains habitat that is critical for the species to survive. We summarised data for all 388 species listed by the end of March 2014, and asked which threats are the most significant, whether the timelines set out in the law are being met, and whether the objectives that are being set are ambitious enough for species recovery.
Human disturbance from recreation, invasive species, and residential or commercial development are the most common threats to species at risk2. This likely reflects the fact that plants are the largest group of species listed under SARA, and listed plants occur in areas of high human activity. Our data show that almost 75% of all species at risk occur along Canada’s southern border, and that over 40% of the species at risk in Canada are plants. To protect at risk plants, we must have specific goals to reduce these threats, but we may not be meeting these objectives.
Many species were automatically listed the same year SARA was enacted, and this created a large backlog of species waiting for recovery strategies to be written. We found that since 2009 there has been steady progress made in completing recovery strategies. However, only around 60% of the 380 species that required recovery strategies by the beginning of 2014 had a finalized recovery strategy. One reason why there are so few strategies is because it takes so long to complete them. By the time most strategies were completed they were more than four years overdue. We predict that at the current rate it will take between six and seven years from the time of listing to the completion of a recovery strategy.
Plants (in which we include vascular plants, mosses and even lichens) fare relatively well under SARA. More plant species than other groups have finalized strategies. However, these strategies are often incomplete and over the years their goals for recovery are progressively becoming less ambitious. Almost 50% of finalized strategies did not designate critical habitat as required. Furthermore, the number of species with recovery objectives that aim to increase the number of individuals, populations, or distribution of a species has steadily declined. Low ambition of these objectives means that the outlook for many species is poor, because by the time they are listed, most species have already undergone large declines in their numbers or substantial reductions in their ranges. Simply stopping declining trends is not real recovery. Recovery is the act of restoring healthy and self-sustaining populations, not merely stalling extinction. After examining the data we collected, we worry that meaningful recovery is not being targeted, and will likely not be achieved.
1 SARA (Species at Risk Act) (2002) An act respecting the protection of wildlife species at risk in Canada. SC 2002, c 29, s 15. Available: http://Laws-lois.justice.gc.ca/PDF/S-15.3.pdf.
2 McCune JL, Harrower WL, Avery-Gomm S, Brogan JM, Csergő A, et al. (2013) Threats to Canadian species at risk: An analysis of finalized recovery strategies. Biological Conservation 166: 254–265 http://dx.doi.org/10.1016/j.biocon.2013.07.006.
Caption 1: A Garry oak savannah is one Canada’s rarest ecosystem and home to many of the plants listed under the Species At Risk Act (SARA). Three multi-species recovery strategies are available for Garry oak ecosystems. These include one for Garry oak woodlands, one for maritime meadows associated with Garry oak ecosystems, and one of vernal pools and other ephemeral wetlands. Photo: Jenny McCune
Caption 2: Carolinian Forest, or Canada’s eastern deciduous forest, is an ecosystem that occurs around Lake Erie that contains many species of plants and animals that occur nowhere else in Canada. The threats to this unique ecosystem include habitat loss to agricultural and residential development. Photo: Jenny McCune
Caption 3: Carl Rothfels, a post-doctoral researcher in UBC’s Biodiversity Research Centre, contemplates the Mexican mosquito-fern (Azolla mexicana). This small fern of wetlands, ponds, and quiet lakes is threatened in Canada by urban and residential development and pollution of its wetland habitat. Mexican mosquito-fern grows in association with the blue-green bacteria that live in cavities on the fern’s leaves. Photo: Marc Johnston
Caption 4: Baikal Sedge (Carex sabulosa) is an at risk plant that occurs in the southern and western Yukon. It is only known from 14 historical sites, but with the help of Aboriginal traditional knowledge other potential sites have been identified and are being surveyed. Baikal Sedge grows only on active sand dunes, and is usually one of the first plants to colonize. Baikal sedge is threatened by invasive species, recreational vehicle use, and residential development. Photo: Syd Cannings
Article by Xin Li lab
Like animals, plants are constantly exposed to bacteria, viruses, and fungi that have the potential to cause diseases. To remain healthy plants possess a complex immune system which relies on immune receptors that are able to recognize pathogens and respond before an infection is established. If a pathogen is able to avoid detection by the immune system, the resulting plant disease can result in dramatic economic losses. An estimated 15% of potential crop output is lost to plant diseases, and periodic disease outbreaks can lead to large-scale social turmoil, such as the Irish potato famine1. Despite the importance of the plant immune system, the molecular details of immune signalling are only partially understood. Thus, the study of plant-microbe interactions is an active area of research, often involving the model plant Arabidopsis thaliana.
Plants have cell-surface receptors to detect common molecules produced by microbes, such as components of bacterial flagella and fungal cell wall polymers. However, many plant pathogens produce effector proteins that hamper this recognition. In response to this, higher plants have evolved Resistance (R) proteins that act as sensors to detect specific effectors. They may bind to effectors and recognize them directly, or they may recognize the changes that the effectors cause on other host proteins. Recognition of the effectors leads to activation of the R proteins, culminating in a defense response that effectively prevents the spread of the pathogen into healthy tissue.
Although plant cells must contain high enough levels of R proteins to quickly recognize invading pathogens, plants must carefully regulate the levels of these proteins in order to optimize growth, because excessive R protein accumulation may activate the defense responses even in the absence of pathogens. This is detrimental to the plant, as exemplified by the snc1 mutant of Arabidopsis identified by UBC professor Dr. Xin Li2,3. The snc1 mutant produces a form of an R protein that is more resistant to protein degradation, and therefore accumulates to higher levels in the plant.
In an attempt to search for genes which are involved in immunity, a forward genetic screen was performed looking for mutants which enhanced the autoimmune phenotype of snc1; this was named the muse (mutant, snc1-enhancing) screen4. From this screen a variety of mutants were identified, many of which play roles in R protein turnover. One of the mutants, muse6, was identified to be a subunit of an N-terminal acetyltransferase (Nat) complex5. N-terminal modification has been a long documented method of proteins regulation in plants as well as other eukaryotic organisms6.
Two recent UBC Botany graduates, Dr. Fang Xu and Dr. Yan Huang, made most of the findings in the story. This discovery led to a detailed study of the N-terminal modifications of SNC1. What was discovered is that SNC1 has a rather unusual pattern of N-terminal modification. The SNC1 protein was found to have alternative initiation, meaning that its translation can begin from either of two adjacent methionines (Met); interestingly, SNC1 appears to be differentially regulated depending on which Met is used as the start codon. If SNC1 translation begins at the first Met it is acetylated by NatA, the Nat complex of which MUSE6 is a component, whereas if it begins at the second MET it is acetylated by a different complex, NatB.
The result of this alternative acetylation has unexpected consequences on SNC1 regulation. The initial screen demonstrated that disrupting NatA caused an increased autoimmune phenotype, and this is because acetylation of the first Met of SNC1 targets the protein for degradation. Alternatively, the acetylation of the second Met by NatB was found to stabilize SNC1. This interesting combination of alterative initiation and differential acetylation has never been reported until now. This novel pattern of alternative acetylation is likely one factor allowing for careful regulation of SNC1 protein levels, and by acting at the level of translation it allows for rapid changes in protein levels during the induction of defense responses.
1. Dangl, J. L., Horvath, D. M. & Staskawicz, B. J. Science 341, 746–751 (2013).
2. Li, X., Clarke, J. D., Zhang, Y. & Dong, X. Mol Plant Microbe Interact 14, 1131–9 (2001).
3. Zhang, Y., Goritschnig, S., Dong, X. & Li, X. Plant Cell 15, 2636–2646 (2003).
4. Huang, Y. et al. Nat. Commun. 4, 2558 (2013).
5. Xu, F. et al. Plant Cell. May 12. pii: tpc.15.00173 (2015). [Epub ahead of print]
6. Gibbs, D. J., Bacardit, J., Bachmair, A. & Holdsworth, M. J. Trends Cell Biol. 24, 603–611 (2014).
Figure 1. A nata mutant, muse6-1, identified from a genetic screen to search for enhancers of snc1.
Figure 2. A working model on the different N-terminal acetylations of SNC1. In wild type plants, SNC1 protein synthesis can be initiated at either the first or the second Met. The peptide translated from the first Met can be acetylated by NatA, which serves as a degradation signal. The peptide translated from the second Met can be acetylated by NatB, where the protein is stabilized by the modification. SNC1 protein homeostasis is maintained by the coexistence of these different protein isoforms. In nata mutants including naa15-1, aminaa15 and aminaa10 plants, almost all the SNC1 peptides are acetylated at the second Met by NatB due to the inefficiency of NatA. The stabilization by acetylation on the second Met leads to increased SNC1 accumulation, partly leading to the autoimmune phenotypes of these plants. In natb plants, as a result of inefficient acetylation of the second Met of SNC1 by NatB that stabilizes the protein, SNC1 protein levels are decreased.
Article by Hélène Sanfaçon
Plant viruses can cause significant economic losses in cultivated plants by inducing visual symptoms that affect plant productivity. However, there are also many viruses that co-exist with their hosts without inducing significant symptoms. Symptom severity is determined not only by the ability of viruses to multiply and to disrupt the physiology of the plant but also by plant defense responses that attempt, sometimes unsuccessfully, to control virus infection.
Our lab, which is located at the Pacific Agri-Food Research Centre in Summerland, BC, and is associated with the Department of Botany at UBC, has been interested in studying how plants and viruses interact and how these interactions influence symptom severity. In particular, we have been studying the molecular mechanisms regulating symptom recovery in Nicotiana benthamiana plants infected with tomato ringspot virus (ToRSV). ToRSV causes economic losses in fruit trees (apple, peach etc…), grapevine and small fruits (raspberry, blueberry etc…) in North America and also infects a wide variety of non-cultivated herbaceous plants such as N. benthamiana. After inoculation with ToRSV, N. benthamiana plants develop necrotic symptoms on the inoculated leaves and on leaves immediately above the inoculated leaves. However, later in infection, plants recover from infection and new leaves emerge that are asymptomatic (often referred to as recovered leaves) (Fig. 1).
In initial studies, we found that the recovered leaves contain viral nucleic acids (RNA in the case of ToRSV) in concentrations similar to those found in symptomatic leaves (1). This left us puzzled: if the virus is still there, why is it no longer inducing symptoms? Basudev (Basu) Ghoshal (UBC Botany Ph.D. student) started in the lab in May 2009, and was determined to get answers. He first showed that symptom recovery is temperature-dependent and occurs at 27oC but not at 21oC (Fig. 2). Although viral RNAs are present in similar levels in symptomatic or recovered leaves, the concentration of viral proteins is much decreased in recovered leaves compared to symptomatic leaves. Further analysis revealed that this is due to a severely reduced rate of translation of the viral RNAs just prior to the onset of symptom recovery (2). This repression of viral RNA translation is not observed in plants grown at 21oC, and as a consequence, viral proteins accumulate to high levels and the plants continue to develop severe symptoms. These results indicate that accumulation of viral proteins, controlled by the rate of translation of viral RNAs, regulates symptom severity.
RNA silencing is a well-characterized plant antiviral response that generally acts by degrading viral RNAs in a sequence-specific manner. RNA silencing is also known to be more active in plants grown at higher temperature. Although RNA silencing has been implicated in other symptom recovery phenotypes, we knew that if it was involved in ToRSV-infected plants, it likely used a mechanism distinct from RNA degradation, since viral RNAs were still present in high concentration in recovered leaves. Basu decided to test the effect of down-regulating the expression of ARGONAUTE (AGO) proteins, which are key enzymes of plant RNA silencing pathways. We were very excited when he discovered that plants deficient in AGO1, one of the 10 plant AGO proteins, did not recover from infection (Fig. 3). In addition, these plants are unable to repress the translation of viral RNAs. Thus, viral proteins accumulate to a high level (2). These results provided strong evidence that translation repression can function as a novel plant antiviral mechanism and that AGO1 is involved directly or indirectly in this mechanism.
Basu defended his thesis in July 2014 and the introduction chapter of his thesis was adapted to be published as an authoritative review on the various RNA silencing mechanisms associated with symptom recovery in virus-infected plants (3). This review, now in press, will be published in conjunction with the 60th anniversary issue of the journal “Virology” and includes a model for the newly-discovered translation repression mechanism. We are hoping that the new insights in the molecular mechanisms regulating symptom recovery will lead to improved plant disease management strategies applicable to cultivated plants in the field.
1. Jovel J, Walker M, Sanfacon H. 2007. Recovery of Nicotiana benthamiana plants from a necrotic response induced by a nepovirus is associated with RNA silencing but not with reduced virus titer. J Virol 81:12285-12297.
2. Ghoshal B, Sanfacon H. 2014. Temperature-dependent symptom recovery in Nicotiana benthamiana plants infected with tomato ringspot virus is associated with reduced translation of viral RNA2 and requires ARGONAUTE 1. Virology 456-457:188-197.
3. Ghoshal B, Sanfacon H. 2015. Symptom recovery in virus-infected plants: Revisiting the role of RNA silencing mechanisms. Virology in press.
Figure 1: Symptom recovery in N. benthamiana plants infected with ToRSV. Inoculated leaves develop symptoms in the form of necrotic rings by five days post-inoculation (5 dpi, left). At 8 dpi, leaves immediately above the inoculated leaves display necrotic symptoms along the veins (centre). At late stages of infection, the plant apparently recover from infection and new leaves are asymptomatic (right).
Figure 2: Temperature-dependent symptomatology in N. benthamiana plants infected with ToRSV. Although necrotic symptoms initially develop on ToRSV-infected plants grown at 21 or 27oC, symptom recovery is only observed at the higher temperature. At 21oC, infected plants become completely necrotic and eventually die (pictures show plants at 20 dpi).
Figure 3: Symptom recovery depends on AGO1. Although wild-type plants recover from infection by 16 dpi at 27oC (top panel), plants that are deficient in AGO1 do not (lower panel). The stunting of AGO1-deficient plants compared to wild-type plants is a well-documented side-effect related to the regulation of plant development by AGO1.
Article by Sandra Lindstrom, Paul Gabrielson, Jeff Hughey, Erasmo Macaya and Wendy Nelson.
This project is not something I ever envisioned. How did someone who is interested in the biogeography and phylogeny of seaweeds occurring on the West Coast of North America end up leading a project that revised the taxonomy of a genus of seaweeds known only from the Southern Hemisphere? It was in fact because of my interest in a genus of predominantly Northern Hemisphere species (Mastocarpus, the Turkish washcloth) that I asked Paul Gabrielson to collect specimens of that genus for me in Chile. What he collected turned out to be something different when I sequenced the specimens. When the sequences were blasted against the GenBank database in 2008, they came out closest to another Northern Hemisphere group I was interested in (the Palmariaceae). Moreover, those sequences represented three different species of Nothogenia fastigiata.
In 2009 I discussed my observations with Max Hommersand and Wendy Nelson, two people with Southern Hemisphere interests, at a conference in Tokyo. Both encouraged me to pursue work on the genus with promises to send me material from their own collections. This resulted in sequences of additional cryptic species from New Zealand and beyond. Since a number of species names had previously been subsumed in Nothogenia fastigiata, it became necessary to sequence the type specimens of these species to determine whether any of these names could be resurrected to apply to the cryptic species I had uncovered. Time to call in the guru of ancient DNA sequencing: Jeff Hughey.
Finally, at a conference in Orlando, Florida, in 2013 I heard a talk by Erasmo Macaya on diversity in Chilean Nothogenia. His results mirrored my own. Paul and I invited Erasmo to join the project, and Erasmo subsequently sent me dozens of Chilean collections to sequence.
In the end, I sequenced the ribosomal ITS regions and the rbcL, psbA and COI genes in up to 56 Nothogenia specimens, plus a few other species that collectors had misidentified as Nothogenia. Together with Jeff’s sequencing of type specimens, we were able to recognize 10 species of Nothogenia in the Southern Hemisphere, including four that were resurrected from previous synonymy with N. fastigiata and one newly described species. Most of these species occur in geographically restricted areas, but N. fastigiata appears to indeed be widespread, with collections verified from its type locality of the Falkland Islands, from Chile, and from Campbell Island south of New Zealand. Another surprise was that Tasmanian N. lingula is more closely related to N. fastigiata and other Chilean species than to the geographically closer New Zealand or South African species. Several Chilean species remain undescribed, and it has fallen to Erasmo to take the lead on these.
Unfortunately, long before this project was completed, my NSERC research funding expired. Thus, most of the expenses associated with sequencing were covered by funds from a Census of Marine Life grant and from my mother. I covered all other expenses myself.
S.C. Lindstrom, P.W. Gabrielson, J.R. Hughey, E.C. Macaya & W.A. Nelson. 2015. Sequencing of historic and modern specimens reveals cryptic diversity in Nothogenia (Scinaiaceae, Rhodophyta). Phycologia 54: 97-108.
Caption: Photos showing the different morphologies of Nothogenia fastigiata (a) and Nothogenia “C” (b), the only species of Nothogenia to co-occur on the same shore in Chile (courtesy of Erasmo Macaya)
Article by Bill Harrower, Lauchlan Fraser and Roy Turkington
Grasslands of British Columbia’s southern interior mountains provide stunning landscapes and host many of the provinces at risk species of plants and animals. Hot dry sagebrush and bunchgrass ecosystems occupy the valley bottoms and grade to the cool wet grasslands with increasing elevation.
The structure of ecosystems varies enormously because of their location and the organisms that live there. The Fraser and Turkington labs have studied the structure and function of many types of ecosystems – deserts, savanna grasslands, boreal forest understory, subtropical forest understory, and grasslands in Yukon and interior British Columbia. Using observations and experiments we have investigated how the plants and animals that live in grasslands interact to produce the abundance and complexity of life we see. One of the major techniques we use is called a removal experiment. We document all the plants and animals in an intact ecosystem and monitor various measures of ecosystem function such as rates of productivity and decomposition, soil nutrient levels and turnover rates. We then remove specific components of the system such as small birds, mice and voles, insects, plant species, and litter to determine how ecosystem composition and function change in response to these removals. In this way we can predict the consequences of disturbances (such as might be associated with climate change or overgrazing) and species loss on the structure and function of grasslands. We have done this research in grasslands having different amounts of rainfall and ranges in temperature. By comparing the effects of species removals in cool wet grasslands to removals in hot dry grasslands, we gain an understanding of the processes that structure ecosystems, promote biodiversity, and ultimately supply humans with the services we need. We found that the removal of song birds and small mammals can have profound effects on the growth and diversity of plants, and that these effects depend on whether we are in a hot dry grassland or a wet cool grassland. Predation by birds and mammals on insects alters the amount of herbivory on plants. When we remove bird and mammals we change insect communities and this increases plant growth in wet cool grasslands or retards growth in hot dry grasslands. Dead plant material, litter, can influence the degree to which birds or mammals influence plants. This is important because the amount of litter in the ecosystems depends on the amount of wildfire and livestock grazing humans allow.
We chose to do this research in grasslands because of their widespread global distribution and because of their great importance to humans. Grasslands filter water, mitigate flooding, clean air, and we often live in or beside them. Grassland ecosystems provide us with much of our food – – crops, forage and livestock. This understanding of grassland ecosystems allows us to conserve grasslands and manage the services they provide to humans.
Article by the Samuels lab
Trees and other plants need strength to stand upright and move water effectively from their roots to their leaves. That strength comes from the cell walls that plant cells build around themselves as they grow. Cell wall material makes up most of the mass of plants. Trees that live for many years form more and more cell wall material every year, while retaining the material from previous years, resulting in considerable accumulations of mass, carbon, and useable materials in the form of timber. Along with cellulose, lignin is an important component of those walls. Lignin is made of large polymer molecules that are made piece by piece through the addition of monomers known as monolignols.
The mystery of monolignol movement
Billions of tons of lignin are made by plants every year, yet how monolignols go from inside of cells, out into the cell wall to form lignin, was a mystery. Monolignols are made inside of cells, but are exported to the cell wall to form lignin. Various explanations of how monolignols cross cell membranes have been proposed over the years, but conflicting and inconclusive evidence meant that the mystery lived on.
The answer: sink-driven diffusion
In his PhD dissertation, Mendel Perkins solves this mystery with a simple explanation: monolignols simply diffuse out of the cell, and quickly get crosslinked into lignin, creating a ‘pull’ for more diffusion. To test this, collaborators at the BC Cancer Research Centre made artificial membranes called liposomes that contained monolignol polymerizing enzymes called laccases. Those liposomes are similar to the lipid particles used to deliver mRNA to human cells in COVID-19 vaccines. The liposomes modelled a living cell but simplified the system to isolate the roles of polymer formation and the resulting diffusion. These experiments demonstrated clearly that monolignols can diffuse across a membrane provided there is a source on one side and a sink consuming them on the other. However, just because something can happen, doesn’t mean that it does happen.
What Perkins needed was a system to test the behaviors of monolignols in living cells. Lignin and related phenolic molecules glow with florescence under ultra-violet light. Using this auto-fluorescence, the lignification process could be watched in living cells using multi-photon microscopy. These experiments showed that monolignols flowed to the cell wall in proportion to the strength of the polymeric lignin forming, consistent with hypothesis of sink-driven diffusion.
This work was a collaborative effort between the Samuels lab in Botany, the Mansfield lab in Forestry, the Castellarin lab in the Wine Research Centre and the Bally lab at the BC Cancer Research Centre.
Perkins M. L., Schuetz M., Unda F., Chen K., Bally M. B., Kulkarni J. A., Yan Y., Pico J., Castellarin S., Mansfield S. D., and Samuels A. L. (2022) Monolignol export by diffusion down a polymerization-induced concentration gradient. The Plant Cell. https://doi.org/10.1093/plcell/koac051
Commentary by Sofia Otero-The law of supply and demand rules monolignol transport The Plant Cell, koac050, https://doi.org/10.1093/plcell/koac050
Picture 1: Cross section of a Black Spruce (Picea mariana) tree. The rings form annually from the accumulation of cell wall material made up of lignin and other materials.
Picture 2: Liposomes containing lignin polymerizing laccase enzymes model the lignifying regions of plant cell walls. Over time, lignin monomers pass through the membrane, reach the laccase enzymes and form polymer. The net effect is a significant one-way movement of monolignol monomers from one side of the membrane to the other.
Picture 3: Autofluorescent signals in Arabidopsis roots allowed for the visualization of the disrupted distribution of lignin and related phenolic molecules in response to disruptions to the polymer forming capacity of cell walls. These observations support the diffusion-based transport hypothesis for lignin monomers.
Originally published by UBC Science on August 2, 2022
For the first time, plant biologists have defined the high-efficiency “hacks” that cannabis cells use to make cannabinoids (THC/CBD). Although many biotechnology companies are currently trying to engineer THC/CBD outside the plant in yeast or cell cultures, it is largely unknown how the plant does it naturally.
“This really helps us understand how the cells in cannabis trichomes can pump out massive quantities of tetrahydrocannabinol (THC) and terpenes—compounds that are toxic to the plant cells at high quantities—without poisoning itself,” says Dr. Sam Livingston, a botanist at the University of British Columbia who led the research.
“This new model can inform synthetic biology approaches for cannabinoid production in yeast, which is used routinely in biotechnology. Without these ‘tricks’ they’ll never get efficient production.”
For centuries, humans have cultivated cannabis for the pharmacological properties that result from consuming its specialized metabolites, primarily CBD and terpenoids. Today, production within Canada’s $2.6 billion-a-year cannabis industry ($20 billion global cannabis market) largely relies on the biological activity of tiny cell clusters, called glandular trichomes, found mainly on the plant’s flowers.
The study, published this week in Current Biology, reveals the microenvironments in which THC is produced and transported in cannabis trichomes, and sheds light on several critical points in the pathway of making THC or CBD within the cell.
Dr. Livingston and co-author Dr. Lacey Samuels used rapid freezing of cannabis glandular trichomes to immobilize the plant’s cellular structures and the metabolites in situ. This enabled them to investigate cannabis glandular trichomes using electron microscopes that revealed cell structure at the nano level, showing that the metabolically active cells in cannabis form a “supercell” that acts as a tiny metabolic biofactory.
Until now, synthetic biology approaches have focused on optimizing the enzymes responsible for making THC/CBD—like building a factory with the most efficient machinery to make as much product as possible. However, these approaches haven’t developed an efficient way to move intermediate substances from one enzyme to another, or from inside the cell to the outside of the cell where final products can be collected. This research helps to define the subcellular “shipping routes” that cannabis uses to create an efficient pipeline from raw materials to end products without accumulating toxins or waste products.
“For more than 40 years, everything that we thought about cannabis cells was inaccurate because it was based on dated electron microscopy,” says Dr. Samuels, a plant cell biologist at UBC. “This work defines how cannabis cells make their product. It’s a paradigm shift after many years, producing a new view of cannabinoid production. This work has been challenging, partly the result of legal prohibition and also due to the fact that no protocol for the genetic transformation of cannabis has been published.”
Jack Reiser Maze was born in San Jose in 1937 and grew up in Hollister, California. His mother, Marguerite, was a schoolteacher and his father, Theodore (a.k.a “Dud”) was a mechanic. Jack did his undergraduate studies at Humboldt State University (College at the time) in northern California, received his MSc (MS) at the University of Washington, working with C.L. (“Hitchy”) Hitchcock, and his PhD at the University of California, Davis, with John Tucker, “Old Man of the Oaks.”
Jack had the good fortune to meet Ellie Bondurant in college, and they were married in 1961. They moved to the University of Toronto, where Jack discovered the contrast between the diverse central California flora (variation within a few miles) and Ontario (where a drive 100-200 miles north revealed little floristic difference). Jack was on a collecting trip in Washington State when he became interested in moving to UBC. He called the Botany Department Head Neil Towers, who told him that Botany needed teaching in the areas of anatomy and ecology. Acceptance was easy because in 1967-68, U of T had not even started to look at renewing contracts for young faculty and other universities were expanding.
On arrival at UBC he found a friendly welcome and a great mentor in Janet Stein who sat and listened and gave good advice. Botany wanted teaching in anatomy, ferns, ecology, and the inevitable assignment to teach Biology 101. Jack noted that he survived from lecture to lecture in Biology 101.
Jack developed his research path by continuing the study of grass development that he began as a student at U of Wash. and later at U of T. He drew on his training in classical taxonomy from his MSc work at U of Wash and the skills in microscopy and anatomy that he learned from the great plant anatomist Katherine Esau during his PhD studies at UC Davis. He looked for ways to study plant embryos along with other reproductive and vegetative structures that could lead to unifying ideas between plant development and evolution.
One of his first collaborators at UBC was Bill Parker (MSc, Forestry 1972; PhD 1975 with Bruce Bohm). They discussed ways to link forms in juvenile and mature true firs in north central BC and how to quantify this variation. Parker’s skill with a rifle allowed him to shoot samples from treetops to collect tips from 30-60 metres above the ground and then make cone measurements from older and younger parts of the same tree. These measurements were subject to more sophisticated analyses when Jack collaborated with Gary Bradfield, who showed many colleagues the value of Principle Components Analysis (PCA). Gary had worked with L. Orloci, a student of forest ecologist V. Krajina.
Cyril (Cy) Finnegan was an embryologist in UBC Zoology who later became Dean of Science. Cy taught Zoo 400, the History and Philosophy of Biology, a course that Jack loved and taught after Cy retired. Jack encouraged students to sit in on Cy’s lectures even if they weren’t taking it for credit – several students and a few faculty took this advice and gained a deeper understanding of the structure of science and its applications in biology. Jack and Cy had many theoretical discussions on development and evolution that over the years led to the publication of a few adventurous papers on the idea of species as a virtual code with Edwina Taborsky, who studied semiotics.
Jack’s interest in the relationship between development and speciation grew deeper after he met systematist Dan Brooks from the Department of Zoology, UBC. Dan was working on a book, Evolution as Entropy, with another systematist and fellow cladist, Ed Wiley, invoking the Second Law of Thermodynamics as the underlying drive for self-organization and the emergent phenomena of ontogenesis and phylogenesis, or speciation, based in part on the chemical observations of Ilya Prigogine and his colleagues. Rob Scagel read several relevant papers by Brooks and found that Dan and Jack agreed that information theory could provide a useful analysis of energy as information that is carried in cell walls as cellulose, for example, and that organisms and species can be viewed as energy systems. Discussions and collaborations between Jack and students Rob Scagel, Kali Robson, and Mishtu Banerjee, led to another question – are populations really the relevant level of organization in evolution? Peter Sibbald added excellent computer skills to the idea that there was much more information in DNA and devised statistical approaches in terms of ‘bit per base’. Most information density was in doublets, he found, and this was a way to ensure information transfer.
Jack’s interest in grasses continued throughout his career. He and Rob Scagel collected specimens of Nelson’s needlegrass (Achnatherum nelsonii) and Richardson’s needlegrass (A. richardsonii) for study. On a field trip near Kamloops in 1983 they were excited to find hybrids between the two and collected them all for their ongoing multivariate studies comparing changing variable correlations in homologous structures.
Species membership in complex organisms can only be expressed through the development of individuals, and this relationship intrigued Jack. He gave a departmental seminar in which he discussed the changes in hybrids compared with the differences between juvenile and adult stages he had been studying with Rob Scagel. He related this to changing organization, emergence and non-Darwinian views of development and speciation. Vladimir Krajina was not amused and told Rob that he was a good botanist, but he had fallen under the evil influences of those who deviated from the standard concepts of fitness and selection in the study of evolution. There was a photo of Jack with a frosted beard taken when he was on sabbatical in Saskatchewan visiting Taylor Steeves, and this was transformed into a logo drawn by a PhD student of Bob Scagel, Jim Markem, with the motto “Evil Influences.” Jack loved it and made it into letterhead – typical Maze sense of humour.
It seemed that this was a good time for another application to NSERC given that in the 1980s DNA content had been described in terms of information. As always Jack persisted in his efforts to raise the importance of “information”, and other projects led to collaborations with Pat Bowen (Agriculture Canada) who linked grape leaf development to temperature. Kali Robson (MSc 1986) was fascinated by Jack’s approaches to quantification and comparison and applied them to Balsamorhiza growth in her MSc and beyond. Jack was also interested in rare grasses, including the diminutive Henderson’s ricegrass, Achnatherum hendersonii. During the 1990s, he and Kali compared the variation in measured structures of this grass with Lemmon’s needlegrass, A. lemmonii, and other widespread species growing nearby. He also worked on rare and common grasses with Jill Rapson, an ecologist from New Zealand. On a collecting trip in eastern Oregon, Jack and Kali came across another little ricegrass that turned out to be a new species. Jack called it A. wallowaensis, naming it for its home in the Wallowa Mountains instead of for an obscure botanist. In 2022, Jack, Cindy Roché and James David published a paper on this rare grass and its lithosol habitat.
Jack taught a variety of courses in Botany and in the Biology Programme as well as in the Arts 1 Programme, where he became good friends with co-instructor Dr. Brandon Konoval from UBC School of Music. Jack believed that students should understand sharing facts, information and the value of correlations. It was one of his goals that they should hear unconventional things. He offered the idea that DNA was the servant of cytoplasm, and not the other way around. He was talking about epigenetics at the level of morphogenesis and structural organization. One of his Arts 1 students accused him of trying to warp their minds; he replied that he was paid to warp young minds. Students loved him for it.
Jack appreciated plants for themselves and spent time in the herbarium with colleagues including V. Krajina (whom he respected despite their disagreements), Kay Beamish and bryologist Wilf Schofield. He collaborated on essays about aesthetics, about the importance of the intrinsic beauty of plants.
Jack’s doctoral research was on oaks, on hybrids between bur oak, Quercus macrocarpa, and Gambel oak, Q. gambelii. He concluded that hybrids persisted as stable populations even after one of the parents had vanished from an area. The cultivated hybrid, Quercus ‘Jack Maze’ is available from commercial nurseries. It is grown in some public parks for its form, colour and drought tolerance.
Students were drawn to Jack for his down-to-earth open-mindedness and his small office was often crowded. Arguments and ideas were illustrated on his little chalkboard, sometimes just for the fun of it. Ellie had Jack invite new students over for dinner, and their home was always open for parties and visitors. Jack’s students and others, including Brian Compton, who he shared with ethnobotanist Nancy Turner, along with Lacey Samuels, Shona Ellis, Naomi, Randy Thompson, Loyal Mehrhoff and Peggy Oyama became lifelong friends
Ellie passed away in 2014, after 53 years of marriage; Jack continued to live alone in their house on West 14th Ave. He enjoyed conversations with Cy Finnegan, and the whole Finnegan family. Jack and Ellie were also close with Bruce and Leslie Bohm and their family. Leslie was a skilled artist and had illustrated many of Jack’s publications. Jack organized several trips on the memorial of Ellie’s death in July to Colockum Pass, outside of Wenatchee, WA, where they had camped and done field work with grasses and conifers. It was their favourite place. These trips ended after 2019, when the Covid 19 pandemic hit, but Jack continued to remember Ellie every July with a small gathering of friends. Another homage to Ellie involved breakfasts with friends at the Sunshine Diner on Broadway.
In fall 2024, Jack had a series of small strokes and a fall that left him unable to walk. He returned home with kind caregivers including Natalie Williams as well as Armanpreet Kaur and her colleagues from ABC Home Support. Although he was bed-bound, Jack continued to write haiku and enjoyed telling stories. He and fellow poet Christine in Wyoming exchanged haiku daily. Nancy Turner and friends on Protection Island would send him inspiring photographs of nature, and he would send a haiku by return email. The week before he died, he had a last haiku published in The Avocet, an on-line poetry forum he had contributed to for many years. On February 26, 2025, Jack had a heart attack at home and died a few hours later at Vancouver General Hospital. Shona Ellis and Lacey Samuels, who supported him in his last months, were with him as he peacefully passed away.
‘Round the pool, Jack.
This obituary was based on Iain Taylor’s History of UBC Botany (unpublished), and recollections from Kali Robson, Rob Scagel, Mishtu Banerjee, Peter Sibbald, Lacey Samuels, and Shona Ellis.
Professor of Teaching Emerita
Student Learning: Transitions to learning in university, student attitudes toward learning in science, student ‘misconceptions’, efficacy of inquiry-based learning, self-regulation of learning.
Intertidal and Plant Ecology: Interactions between native and introduced species.
Academic History
Contact Information
nomme@zoology.ubc.ca
Research Interests
My role in the Biology Program is to teach. This encompasses the many elements of teaching biology to undergraduate students; curriculum design and implementation, course administration and coordination, and graduate student preparation for teaching.
I have adopted a scholarly approach to teaching and have initiated research projects to investigate elements of student learning. My research interests include investigating the effectiveness of curriculum revisions and active-learning strategies on student attitudes and student adjustment to higher education. In conjunction with colleagues we have developed assessment tools designed to measure changes in student attitudes towards Biology as a Science and concept inventories to measure learning gains in fundamental concepts (Questions for Biology Q4B http://q4b.biology.ubc.ca/). The results of these investigations have been shared at conferences, Teaching and Learning meetings and are at various stages of publication.
Teaching Interests
I am very interested in student learning and have been involved in many aspects of undergraduate teaching and student learning.
My role in the Biology Program has been to teach, primarily First-Year students, to pursue teaching and learning research interests, and to facilitate the training of graduate student Teaching Assistants. Using an evidence-based approach I have investigated best-practices in teaching that facilitate student transition to learning in university. Areas of research include:
1. Investigation and measurement of student attitutdes towards Science.
2. Development of active-learning teaching strategies and field experiences.
3. Measurement of conceptual learning (Q4B https://q4b.biology.ubc.ca/).
4. The intersection of self-regulation and inquiry-based learning.
I have also contributed to the development of a series of Teaching Assistant training workshops (BioTAP https://blogs.ubc.ca/biotap/) and continue to participate in the workshops promoting best practices in teaching and learning.
Selected Publications
Jeffery, E., Nomme, K., Deane, T.D., Pollock, C. and Birol, G. (2016). Investigating the Role of an Inquiry-Based Biology Lab Course on Student Attitudes and Views toward Science. CBE-Life Sci. Educ. 15: ar 61 DOI:10.1187/cbe.14-11-0203.
Deane, T.D., Nomme, K., Jeffrey, E., Pollock, C. and Birol, G. (2016). Development of the Statistical Reasoning in Biology Concept Inventory (SRBCI). CBE – Life Sci. Educ. 15, no. 1, ar 5 DOI: 10.1187/cbe.15-06-0131.
Nomme, K.M., M. Moussavi, and C. Pollock. (2015). Twenty years of inquiry-based labs: Does it work? Pages 35-37 in Tested Studies for Laboratory Teaching, Volume 36 (K. McMahon, Editor). Proceedings of the 36th Conference of the Association for Biology Laboratory Education (ABLE). 3 pages. HTTP://www.able.wcb.org/volumes/vol-36/v36reprint.php?ch=
Nomme, K. and Birol, G. (2014). “Course Redesign: An Evidence-Based Approach,” The Canadian Journal for the Scholarship of Teaching and Learning: Vol 5: 1, Article 2. DOI: http://dx.doi.org/10.5206/cjsotl-rcacea.2014.1.2 Available at: http://ir.lib.uwo.ca/cjsotl_rca cea/vol5/iss1/2
Deane, T.D., Nomme, K., Jeffrey, E., Pollock, C. and Birol, G. (2014). Development of the Biological Experimental Design Concept Inventory (BEDCI). CBE – Life Sci. Educ. 13: 540-551.
Recent Conference Presentations and Posters
Nomme, K. Overview of BIOL 140. First-Year Educators’ Symposium: Teaching Strategies Focus on Undergraduate Research Experience as a Vehicle to Teach Communication Skills: How and Why. UBC (January 2018), Local.
Nomme, K. Overview of BIOL 140 Investigations in Biology in First-Year. Exploring the CURE: A UBC-based symposium bringing together life science educators with shared interests in Course-based Undergraduate Research Experiences. (May 2017), Local.
Kalas, P., Nomme, K., O’Neill, A., Pollock, C., Suarez, A. Workshop: Using Active Learning Techniques for Teaching Effective Instructional Strategies. BC BIO :Supporting Student Growth, SFU (May 2017), Local.
Nomme, K., Schimpf, N., Kalas, P., Assessing Student Learning Following Course Revision. The Western Conference on Science Education (WCSE): Embracing Change. London, Ontario (July 2017) National.
Nomme, K., Kalas, P., Schimpf, N. Using Concept Assessments to Evaluate Student Learning Gains and Course Curriculum Revision. 6th International Assessment in Higher Education conference (AHE). Manchester, UK (June 2017) International.
Nomme, K., O’Neill, A., Pollock, C. Using Active Learning Techniques for Teaching Effective Instructional Strategies. Northwest Biology Instructors Organization Annual Conference (NWBIO) Tacoma Washington (May 2017) International.
Kalas, P., Schimpf, N., Nomme, K. Standardizing Scientific Conventions Across the Biology Program, (August 2016), Local.
Schimpf, N., Nomme, K., Kalas, P. BIOL 140 Renewal: Responding to Student Feedback. UBC Sceince Education Open House (April 2016), Local.
Nomme, K. Kalas, P., Schimpf, N., Engaging and Learning in Authentic Environments – Does Scaffolding of the Inquiry Process Enhance Student Learning. UBC-O 12th (May 2016) Local
Nomme, K., Kalas, P., Schimpf, N., Developing Scientific Writing Skills, NWBIO Eugene Oregon, (April 2016), International.
Professor Emeritus
Instructor Emeritus
Contact Information
ellenr@mail.ubc.ca
Professor Emeritus
Contact Information
iain.taylor@botany.ubc.ca