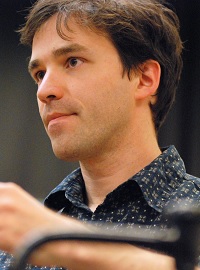
Professor
CRC Tier 1
Academic History
- B. Sc. (1992), Genetics, University of Western Ontario,
- Ph.D. (1996), Biochemistry, Dalhousie University.
- Visiting Scientist, Plant Cell Biology Research Centre, University of Melbourne, (1996-1998),
- Postdoctoral Fellow, Indiana University (1998-1999).
My Links
- Visit the Keeling Lab Home Page
Contact Information
- pkeeling@mail.ubc.ca
- Office: 604-822-4906
- Office Room 341/Lab 385 Biodiversity Building
- Lab Phone: 604-822-2845
Research Interests
Research in the lab is generally related to the molecular evolution and cell biology of eukaryotes, in particular the protists (i.e., eukaryotes that are not animals, fungi, or plants). Protists are mostly single celled organisms, but are many are extremely complex and sophisticated despite their small size. Protists also represent the greatest part of eukaryotic diversity, although most protists groups are very poorly studied, especially at the molecular level. We use molecular biology, microscopy, and increasingly use genome wide analyses such as EST sequencing and genome sequence surveys to study a number of questions in different lineages of protists. Much of our research focuses on cellular organelles, in particular mitochondria and plastids. These organelles originated by endosymbiosis, or the uptake and retention of a bacterium (the endosymbiont) by a eukaryote (the host). In the case of mitochondria this involved an alpha-proteobacterium and took place around the origin of eukaryotes. Plastids originated more recently from a cyanobacterium. Plastids have also spread between eukaryotic lineages by a process called secondary endosymbiosis. In this case, a plastid-bearing alga is itself taken up by another eukaryote, and its photosynthetic apparatus is retained by this new, secondary, host. Another major focus of the research is the transition of free living organisms to parasitism and how this affects their organelles and metabolism. Below are short descriptions of several projects currently underway.
For current research visit this link: http://www3.botany.ubc.ca/keeling/research.html
Team Members
For current team members visit this link: http://www3.botany.ubc.ca/keeling/people.html
Selected Publications
Husnik, F., Tashyreva, D., Boscaro, V., Georde, E. E. Lukes, J., and Keeling, P. J. 2021. Bacterial and archaeal symbioses with protists. Curr. Biol. 31, PR862-877.
Mathur, V. Wakeman, K. C., and Keeling, P. J. 2021. Parallel functional reduction in the mitochondrion of apicomplexan parasites. Curr. Biol. 31, P2920-2928.
George, E. E., Husnik, F., Tashyreva, D., Prokopchuk, G., Horak, A., Kwong, W.K., Lukes, J., Keeling, P. J. 2020. Highly-reduced genomes of protist endosymbionts show evolutionary convergence. Curr. Biol., S0960-9822, 31703-8.
Tikhonenkov D. V. , Mikhailov K. V. , Hehenberger E., Karpov S. A. , Prokina K. I. , Esaulov A. S. , Belyakova O. I. , Mazei Y. A. , Mylnikov A. P. , Aleoshin V. V. , and Keeling P. J. 2020. New Lineage of Microbial Predators Adds Complexity to Reconstructing the Evolutionary Origin of Animals. Curr. Biol., 30, 4500-4509.
Keeling, P. J. 2019. Combining morphology, behaviour, and genomics to understand the evolution and ecology of microbial eukaryotes. Phil. Trans. Roy. Soc. B., 374, 20190085.
Hehenberger, E., Gast, R. J., and Keeling, P. J. 2019. A kleptoplastidic dinoflagellate and the tipping point between transient and fully integrated plastid endosymbiosis. Proc. Natl. Acad. Sci., USA, 116, 17934–17942.
Keeling, P. J., and Burki, F. 2019. Progress towards the Tree of Eukaryotes. Curr. Biol., 29, R808-R817.
Mathur, V., Kolísko, M., Hehenberger, E., Irwin, N. A. T., Leander, B. S., Kristmundsson, Á. Freeman, M. A., and Keeling, P. J. 2019. Multiple Independent Origins of Apicomplexan-Like Parasites. Curr. Biol., 29, 1-6.
Gawryluk, R. M. R., Tikhonenkov, D. V., Hehenberger, E., Husnik, F., Mylnikov, A. P., and Keeling, P. J. 2019. Non-photosynthetic predators are sister to red algae. Nature, 572, 240-243.
Kwong, W. K., del Campo, J., Mathur, V., Vermeij, M. J. A., and Keeling, P. J. 2019. A widespread coral-infecting apicomplexan with chlorophyll biosynthesis genes. Nature, 568, 103-107.
For past and current publications visit this link: http://www3.botany.ubc.ca/keeling/publications.html